INTRODUCTION
Polyploidy, such as triploidy or tetraploidy, is a condition with increased whole chromosome sets in cells, and it is an important topic in cell biology. This process typically occurs during endomitosis or cell fusion and results in terminally differentiated cells, such as megakaryocytes or embryonic trophoblasts (Edgar & Orr-Weaver, 2001). In particular, tetraploidy is thought to arise from multipolar mitosis, progressive chromosomal loss, and chromosome mis-segregation during mitosis. Subsequently, tetraploid cells give rise to aneuploid daughter cells, because of cytokinesis failure ADDIN EN.CITE.DATA (Ganem et al., 2007; Lv et al., 2012). Also, tetraploidy has a high probability of possessing more than double the amount of spontaneous DNA damage and hence, an increased requirement for DNA repair processes ADDIN EN.CITE.DATA (Mable & Otto, 2001; Storchova & Kuffer, 2008). Even if the amount of DNA damage does not increase significantly, the processes for repair might be less efficient in tetraploidy. Some studies have described that both yeast and mammalian tetraploid cells appear to be more sensitive to DNA-damaging agents than euploid cells ADDIN EN.CITE.DATA (Hau et al., 2006; Storchova et al., 2006).
It has been recently suggested that tetraploidy, like polyploidy, is detrimental to early mammalian development ADDIN EN.CITE.DATA (Imai et al., 2015; Kawaguchi et al., 2009). The cell cycle in a tetraploid mouse embryo is prolonged, and it induces a reduction in the number of cells and results in malformation and abnormal cessation of embryonic development (Koizumi & Fukuta, 1995). Actually, polyploid cells are frequently found in tumors of all stages, and several reports support the idea that tetraploid cells occur as an early step in tumor formation (Storchova & Kuffer, 2008) and cancer ADDIN EN.CITE.DATA (Mazumdar et al., 2006; Duelli et al., 2007). For this reason, it has been suggested that polyploidy is formed from spontaneous miscarriages and could be used as a biomarker for the diagnosis of cancer ADDIN EN.CITE.DATA (Mandrioli et al., 2016). In addition, a tetraploid human is thought to be a rare clinical case that results in high intrauterine lethality in humans, because of a genetic aberration (it is found in 1-2% of early miscarriages); only a few clinical reports of infants diagnosed with tetraploidy are available. In fact, the most common outcome of the tetraploid human fetus is miscarriage, and even if the pregnancy reaches full term, the infant dies shortly after birth (Bothur-Nowacka et al., 2013). Most delivered babies have died between birth and one year of age. The oldest recorded non-mosaics tetraploid patient was 26-months-old (Guc-Scekic et al., 2002), while another female was at least 22-months-old (Lafer & Neu, 1988). Interestingly, in humans, mosaic polyploidy in some parts of specific tissues occurs more frequently than polyploidy in the whole body. Generally, mosaic polyploidy may affect cell development. Also, it has been recently suggested that established tetraploidization in oncogenes is linked to tumorigenic potential (Storchova & Kuffer, 2008). In addition, the spontaneous tetraploidization of primary cells from patients diagnosed with Gardner syndrome was observed several decades ago (Danes, 1976). On the other hand, some reports have suggested that the relatively frequent presence of polyploid cells in normal tissues (0.5-20% depending on the tissue) brings some advantages for the function of tissue differentiation or for functional adaptation (Biesterfeld et al., 1994). However, there is a limitation in the study of polyploidy in the human system, because polyploid human and mammals are very rare cases ADDIN EN.CITE.DATA (Imai et al., 2015), although it is very common among plants, fish, and amphibians in nature. So, in the present study, we have examined the characteristics and function of tetraploid SCNT-hESCs, and believe that this could be developed as a research model for tetraploid cells that exist in human tissues.
MATERIALS AND METHODS
The study protocol (CHA001) was approved by both the CHA Regenerative Medicine Institute (CHARMI) Stem Cell Research Oversight (SCRO) Committee and the Essex Institutional Review Board (EIRB). Ovarian stimulation protocol of anonymous egg donors was followed previous report ADDIN EN.CITE.DATA (Chung et al., 2014). The collected cumulus-oocytes complexes (COCs) were denuded with 50-80 IU/mL hyaluronidase (Sigma-Aldrich) within 1-2 hours after retrieval. Then, they were kept either in Cleavage medium supplemented with 10% serum substitute supplement (SSS; Cooper Surgical) or Global medium 10% (IVF Online) until use.
Human dermal fibroblast (DFB) cells were obtained from normal healthy male volunteers (46 XY). Donor was 35 years old (DFB-1). Small pieces of skin from the inner forearm (0.5 cm × 0.3 cm) were biopsied under local anesthesia and washed 3 times in PBS supplemented with an antibiotic/antimycotic solution (anti-anti 1×, Invitrogen) to remove any possible contaminants. Nuclear donor cell preparation and characterization was followed previous report ADDIN EN.CITE.DATA (Chung et al., 2014).
Human tetraploid SCNT-hESCs can be acquired during traditional SCNT procedure under an inverted microscope equipped with a Polscope (Oosight, Cambridge Research & Instrumentation, UK) to visualize the spindle complex (Fig. 1). All MII oocytes were pre-incubated in Quinn’s Advantage medium with HEPES (Cooper Surgical) containing caffeine (1.25 mM) and 0.5 μg/mL cytochalasin B for 5 minutes. For the enucleation, a holding pipette was used to rotate each oocyte so that the spindle complex was positioned between 5 and 6 o’clock. The zona pellucida below the spindle was cut by a “knife pipette” and a small volume of cytoplasm containing MII spindle was extruded by applying a gentle squeezing force. After the removal of spindle complex, an isolated DFB cell was aspirated into an insertion pipette, transferred, resuspended in a drop containing HVJ-E extract (Isihara Sangyo Kaisha, Japan), and inserted into the perivitelline space of the enucleated oocytes. Differ from traditional SCNT procedure, tetraploid SCNT-hESCs were generated by refusion of remained 1st polar body during the procedure (Fig. 1B).
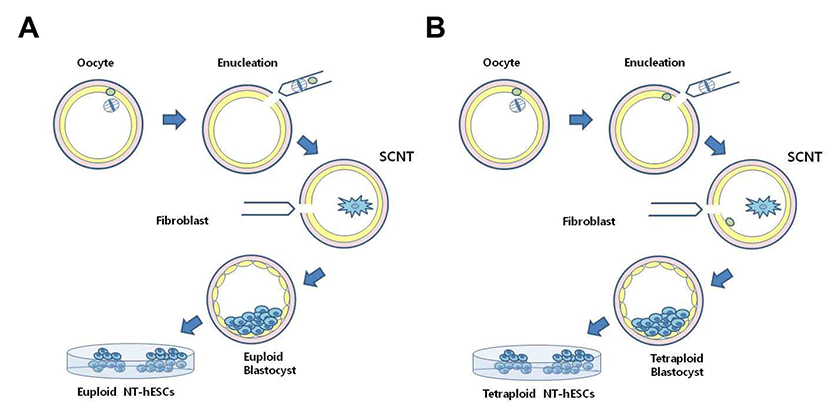
Once cell fusion was confirmed, the reconstructed eggs were transferred into Quinn's cleavage medium 10% SPS, and incubated for 2 hours before activation in an incubator with atmosphere of 5% CO2 / 5% O2 / 90% N2 at 37℃. Activation of the reconstructed eggs was carried out using ionomycin (5 μM, 5 min) and 6-dimethyl aminopurine (6-DMAP, 2 mM, 4 hrs) in Cleavage medium 10% SPS (Cooper Surgical). The cloned embryos were cultured in Global 10% FBS medium containing TSA (10 nM, Sigma) for 12 hrs after activation, whereupon embryos were transferred to Global 10% FBS without TSA and culture. The culture medium was switched to G2 medium supplemented with 10% HSA (Vitrolife) at day 2 and embryos were cultured up to blastocyst by day 6.
After removal of the zona pellucida with acidic Tyrode's solution, whole blastocysts (without removing trophectoderm) were plated onto mitotically-inactivated mouse fibroblasts (MEFs, Global Stem Inc. Rockville, MD) in knockout DMEM-based human ESC derivation medium composed of knockout-DMEM supplemented with Knockout Serum Replacement (10% SR, Invitrogen), FBS (10% Hyclone), bFGF (30 ng/mL), and human LIF (2,000 units/ mL, Sigma-Aldrich) (Chung et al., 2008).
Karyotyping for both NT-cell lines were performed using a standard protocol. Metaphase spreads were stained with a GTG (G-bands by trypsin using Giemsa)-banding technique and 20 metaphases were analyzed and karyotyped by two cytogenetics experts. The ideogram was produced by the Ikaros karyotyping system (MetaSystems, Germany).
Nuclear DNA genotyping of NT-ESCs, donor fibroblasts, and donor oocytes was performed by using AmpFlSTR® identifier kit (Applied Biosystems). PCR reactions were performed according to the manufacturer’s protocol. The PCR products were loaded on ABI 3130 genetic analyzer (Applied Biosystems) and analyzed by the GeneMapper®ID Software v3.2.1 (Applied Biosystems).
To confirm hESC-specific marker expression, tetraploid SCNT-hESCs were cultured with mitomycin-C-treated MEFs on a cover slip. After 48 hrs of culture, the cells were fixed with 4% paraformaldehyde at 4℃ for overnight washed 1 × phosphate buffered saline (PBS, Hyclone) each 15 min washing. After permeabilization by 0.1% Triton-X 100 in PBS for 10 min and blocking with protein block RT for 60 min, the cells were incubated 4℃ for overnight with primary antibodies against OCT-3/4 (SantaCruz sc-5279, 1: 100), SSEA-4, TRA 1-60 and TRA-1-81 (Millipore MAB 4304, MAB4360, MAB4381, 1:100), and Alexa Flour 555 goat anti-mouse IgG antibody (Molecular probes A21424, 1:200) for 1hr at room temperature and All samples were counterstained with 1 μg/mL DAPI (Sigma-Aldrich) for 15 min at room temperature and mounted using vector shield mounting medium (Vector laboratories, Inc,. Burlingame, CA). Sample images were captured with a Axio Imager 2 (Carl Zeiss, Jena, Germany).
The differentiation capacity of tetraploid SCNT-hESCs line was confirmed by EB formation in vitro For EB formation, tetraploid SCNT-hESCs were culture in suspension without human bFGF for 2 weeks, and fixed with 4% paraformaldehyde at 4℃ for overnight after 1.5% Agarose embedding and re-fixed with 4% paraformaldehyde at 4℃ for overnight After washing, the tissues were dehydrated with a 70%, 80%, 90%, 95% and twice 100% alcohol series and twice 100% Xylenec (Clearing), then embedded in paraffin blocks. The paraffin-embedded blocks were serially sectioned at 4 mm. Four-micrometer sections of the paraffin-embedded tissue arrays were deparaffinized, rehydrated in a graded series of alcohol and microwave-treated for 30 min in a target retrieval solution (Dako North AmeriInc., Carpinteria, CA). This process was repeated three times. Sections were blocked with protein blocking solution (Dako) for 1 hr at room temperature. Then, slides were stained overnight at 4℃ with primary antibodies, then the differentiation of EBs into three-germ layers was confirmed by immunohistochemistry. Antibodies against AFP (Alpha-1-Fetoprotein; Dako A0008, 1: 100) for endoderm, 'aSMA (alpha smooth muscle actin; abcam ab5694, 1:100), for medoderm, and Tuj1 (B-Tubulin; Covance PRB-435P, rabbit, 1:100), for ectoderm were used. A Alexa Flour 555 goat anti-rabbit IgG antibody (Molecular probes A21420) was used as a secondary antibody diluted to 1:100 with DPBS (Hyclone) for 1hr at room temperature in dark. All samples were counterstained with 1 μg/mL DAPI (Sigma-Aldrich) diluted 1: 500 with DPBS (Hyclone) for 15 min at room temperature and mounted using vector shield mounting medium (Vector laboratories, Inc,. Burlingame, CA). Sample images were captured with a Axio Imager 2 (Carl Zeiss, Jena, Germany).
The differentiation capacity of tetraploid SCNT-hESCs line was confirmed by, approximately 1×105 of undifferentiated tetraploid SCNT-hESCs were injected into the testicle of a NOD/SCID male mouse. For each tetraploid SCNT-hES cell line, at least 3 animals were used. After 14~16 weeks, teratomas were excised, fixed in 4% paraformaldehyde at 4℃ for 2 week, trimming after re-fixed with 4% paraformaldehyde at 4℃ for 1 week after washing, the tissues were dehydrated with a 70%, 80%, 90%, 95% and twice 100% alcohol series 1 hr at room temperature and twice 100% Xylene, then embedded in paraffin blocks. The paraffin-embedded blocks were serially sectioned at 4 μm. Four-micrometer sections of the paraffin-embedded tissue arrays were deparaffinized, rehydrated in a graded series of alcohol and microwave-treated for 30 min in a target retrieval solution (Dako North AmeriInc., Carpinteria, CA). This process was repeated three times. Sections were blocked with protein blocking solution (Dako.) for 1hr at room temperature. Then, slides were stained overnight at 4℃ with primary antibodies, then the differentiation of teratoma formation into three germ layers was confirmed by immunohistochemistry.
Antibodies against Tuj1 (B-Tubulin; Covance PRB-435P, rabbit, 1:100), for ectoderm were used. A Alexa Flour 555 goat anti-rabbit IgG antibody (Molecular probes A21420) was used as a secondary antibody diluted to 1:100 with DPBS for 1hr at room temperature in dark. All samples were counterstained with 1 μg/mL DAPI (Sigma-Aldrich) diluted 1: 500 with DPBS for 15 min at room temperature and mounted using vector shield mounting medium. Alcian Blue stain for Cartilage and Masson`s trichrome stain for medoderm and periodic acid-schiff (PAS) stain for Secretory epithelium (endoderm) staining Sample images were captured with a Axio Imager 2 (Carl Zeiss, Jena, Germany).
To confirm hESC-specific marker expression, RT-PCR was performed using primer sets (SOX2, F: 5’ - agc tac agc atg atg cag ga - 3’, R: 5’ - ggt cat gga gtt gta ctg ca - 3’, 125 bp; OCT3/4, F: 5’- gca att tgc caa gct cct gaa gca g - 3’, R: 5’ - cat agc ctg ggg tac caa aat ggg g - 3’, 536 bp; NANOG, F: 5’ - tga acc tca gct aca aac ag - 3’, R: 5’ - tgg tgg tag gaa gag taa ag - 3’, 153 bp; GAPDH, F: 5’ - aga agg ctg ggg ctc att tg - 3’, R: 5’ - agg ggc cat cca cag tct tc - 3’, 258 bp). And to confirm the three germ layers differentiation in hEB, used primer sets for ectoderm (SOX1, F: 5’ - gtg tcc aat tgt tgg cat cta ggt c - 3’, R: 5’- caa gga aat aag gtg gtt gga gca c - 3’, 202 bp; NEUROD1, F: 5’ - gtt ctc agg acg agg agc ac - 3’, R: 5’ - tgg aca gct tct gcg tct ta - 3’, 333 bp), mesoderm (ACTC1, F: 5’ - cat cct gac cct gaa gta tcc cat c - 3’, R: 5’ - ccc tca tag atg ggg aca ttg tga g - 3’, 315 bp; HAND1, F: 5’ - aag aga acc aga cgc agg aa - 3’, R: 5’ - ggc agg atg aac aaa cac ct - 3’, 263 bp), endoderm (AFP, F: 5’ - gaa atg act cca gta aac cct ggt g - 3, R: 5’ - aga ctc gtt ttg tct tct ctt ccc c - 3’, 400 bp; SOX17, F: 5’ - cgc acg gaa ttt gaa cag ta - 3’, R: 5’ - aaa aca cac cca gga caa ca - 3’, 510 bp), and control (GAPDH, F: 5’ - aga agg ctg ggg ctc att tg - 3’, R: 5’ - agg ggc cat cca cag tct tc - 3’, 258 bp).
RESULTS
After the SCNT procedure, reconstructed egg were cultured, formed early blastocysts at Day 5, and further developed to hatching blastocysts at Day 6. The hatched blastocyst made an initial outgrowth, resembling an ESC colony, within 3 days and eventually resulted in a stable hESC line (Fig. 1). Different from several diploid SCNT-derived hESCs (SCNT-hESCs), two tetraploid SCNT-hESCs (lines CHA-NT1: 91, XXXY,-6, t[2:6]; CHA-NT3: 92,XXXY,-12,+20) were generated and confirmed by karyotyping (Fig. 2). As the spindle apparatus of MII oocytes was clearly evident in the Polyscope image of enucleation during SCNT, it was suggested that tetraploidy may be caused by reintroduction of the remaining 1st polar body during the SCNT procedure (Fig. 1B). In fact, nuclear DNA genotyping, using the eight Short Tandem Repeat (STR) markers, confirmed that the genotypes of both the CHA-NT1 and CHA-NT3 tetraploid SCNT-hESCs lines included their respective nuclear donor (DFB-1) and oocyte donor genotypes (Table 1).
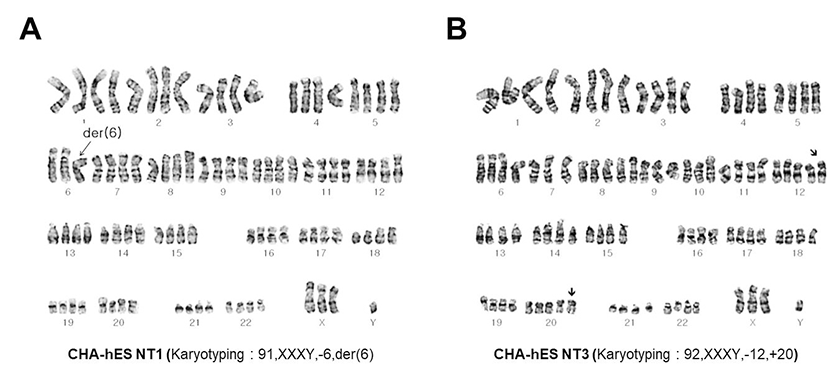
The growth of tetraploid SCNT-hESCs was similar to that of normal diploid SCNT-hESCs (CHA-NT2 and CHA- NT4) (CHA-NT2 and CHA-NT4) ADDIN EN.CITE.DATA (Chung et al., 2014). Also, their colonies and tetraploidy were well maintained after at least 30 manual passages, although a tendency for slow growth during culture was shown. However, a small number of aneuploid cells was sometimes observed during passaging (data not shown).
To characterize the pluripotency of tetraploid SCNT-hESCs, we first analyzed expression of hESC-specific markers. CHA-NT1 and CHA-NT3 hESCs showed the expression of pluripotency genes, OCT-4, SSEA-4, TRA- 1-60, and TRA-1-81, and the RT-PCR results for OCT-4, NANOG, and SOX2 confirmed expression (Fig. 3).
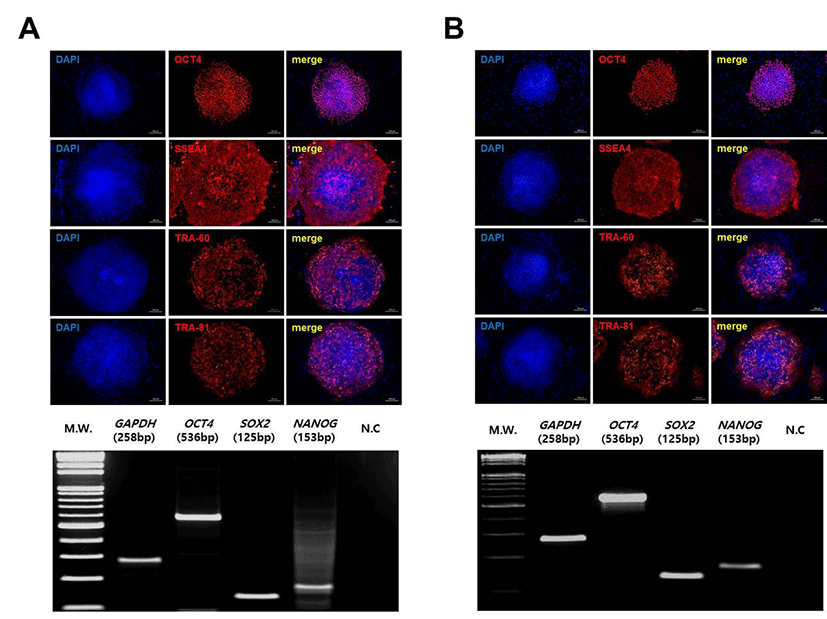
The differentiation capacity of the tetraploid SCNT-hESCs lines was confirmed by EB formation in vitro and teratoma formation in vivo. As shown in Fig. 4, EBs from CHA-NT1 and CHA-NT3 hESCs showed the expression of AFP (a marker for the endoderm), aSMA (a marker for the mesoderm), and Tuji (a marker for the ectoderm). Also, the teratoma formation assay demonstrated that the tissues of the three germ layers (secretory epithelium as the endoderm, muscle fibers and cartilage as the mesoderm, and neural rosette as the ectoderm) grew out from the CHA-NT1 and CHA-NT3 hESCs (Fig. 5).
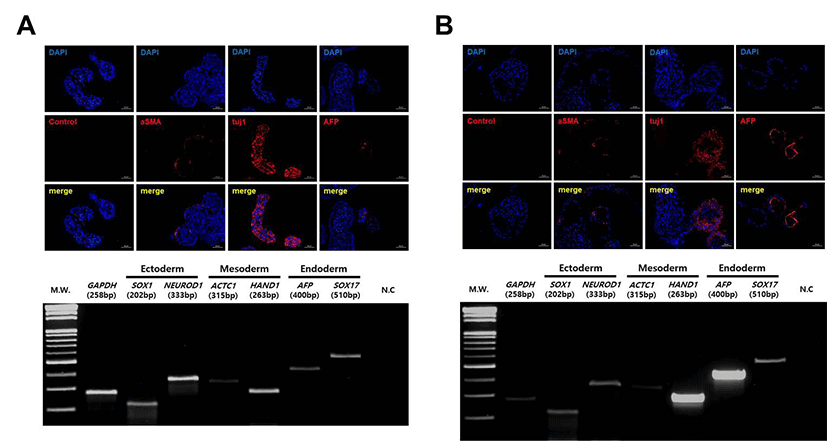
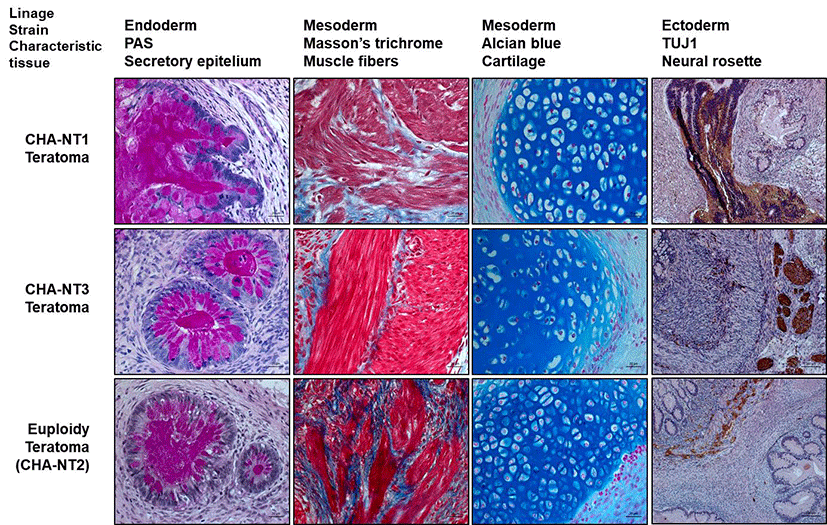
DISCUSSION
In the present study, we have analyzed the characteristics and differentiating potentials of tetraploid SCNT-hESC lines established with traditional SCNT protocols ADDIN EN.CITE.DATA (Chung et al., 2014). Genetic evidence, such as karyotyping and analysis of STR markers, verified the tetraploidy of CHA-NT1 and CHA-NT3, even though CHA-NT1 has one triple (– chromo. 6) and CHA-NT3 has two pentaploid (+ chromo. 12, 20) chromosomes. In fact, the genotypes suggested that CHA-NT1 and CHA-NT3 tetraploid SCNT-hESCs lines may have both genotypes of their respective nuclear and oocyte donors. Therefore, these results may suggest that both tetraploid SCNT-hESC lines were generated by reintroduction of the remaining 1st polar body during the SCNT procedure.
Studies on the characterization have shown that our tetraploid SCNT-hESCs lines expressed the pluripotency markers OCT-4, SSEA-4, TRA-1-60, and TRA-1-81. In addition, immunostaining of AFP, aSMA, and tuj1 in EBs derived from tetraploid SCNT-hESC lines confirmed their potential to differentiate into all three germ layers. Also, the three germ layers were clearly visible in the CHA-NT1 and CHA-NT3 teratomas: secretory epithelium (endoderm), muscle fibers and cartilage (mesoderm), and neural rosette (ectoderm). Also, we have found that tetraploid SCNT-hESC lines maintained their ploidy well and retained stemness after passaging. Therefore, these characteristics support the idea that our tetraploid SCNT-hESCs lines could be useful for the study of tetraploid cells and tissues, because they are very similar to those of euploid SCNT-hESCs (CHA-NT2 and CHA-NT4) ADDIN EN.CITE.DATA (Chung et al., 2014). In fact, in nature, polyploidy is very common among plants, fish, and amphibians, while polyploidy in mammals is a very rare case ADDIN EN.CITE.DATA (Imai et al., 2015). Besides, there are several tissues with tetraploidy in mammals, including human beings. Taken together, this study identified that tetraploidy does not affect proliferation and the differentiating potential in human SCNT-hESCs. So, tetraploid SCNT-hESC lines may have the effect and enable the investigation of the characteristics of polyploid cells in humans. Also, tetraploid SCNT-hESC lines could be a useful model for polyploidy examination.
Meanwhile, in the present study, our tetraploid hESCs have shown a tendency for slow growth in stem cell culture. It has been considered that tetraploidy may interfere with cell division. In fact, it has been reported that tetraploid cells have some problems in the developmental process and growth retardation, due to DNA damage and cellular metabolic stress ADDIN EN.CITE.DATA (Storchova & Pellman, 2004; Ganem et al., 2007). Also, tetraploid cells are highly unstable and prone to transformation. Therefore, tetraploid SCNT-hESC would also be a good research tool for developmental interference in pathogenic cells with abnormal ploidy, such as cancer cells.