INTRODUCTION
Female sex steroid hormones are synthesized in the mammalian ovary. They regulate many homeostatic functions in their target organs (Channing et al., 1980; Adashi, 1994). Among those targets, the uterus is located the closest to the ovary and is directly controlled by these hormones, representatively 17β-estradiol (E2) and progesterone (P4) (Kelleher et al., 2019). For the E2 production, androgens such as androstenedione (A4) and testosterone (T) are synthesized in theca cells that are surrounded by follicles, followed by their conversion to E2 by aromatase activation in granulosa cells of the antral follicles (Hillier et al., 1994). Two pituitary gonadotropins, luteinizing hormone and follicle-stimulating hormone, stimulate the androgen production in theca cells and E2 production in granulosa cells, respectively (Leung & Armstrong, 1980; Hillier et al., 1994). E2 production occurs in the antral follicles whereas P4 is synthesized in luteal cells of the corpus luteum (CL) (Wallach et al., 1996; Devoto et al., 2002).
The ovarian steroidogenesis is largely similar to that occurred in testicular Leydig cells and adrenal cortical cells (Miller & Auchus, 2011). Cholesterol intake and translocation and subsequent pregnenolone synthesis takes place in mitochondria in both theca cells and luteal cells. Cholesterol translocation to the inner mitochondrial membrane is achieved by the steroidogenic acute regulatory (StAR) protein and conversion of cholesterol to pregnenolone is achieved by cytochrome P450 side-chain cleavage (P450scc) (Miller, 2007). Then, pregnenolone is converted to P4 in the endoplasmic reticulum by activation of 3β-hydroxysteroid dehydrogenase (Miller, 2008). In theca cells, P4 is further processed to A4 or T by cytochrome P450 17β-hydroxylase (CYP17) whereas it is the final product in CL as luteal cells lack CYP17 (Stocco et al., 2007; Miller, 2008).
Globally, bisphenol A [BPA; 2,2-bis-(4-hydroxyphenyl)propane] is still being used in large quantities as a plasticizer in production of polycarbonate plastics. It can leach from the lining of metal food cans, polycarbonate baby bottles, dental sealants, polyvinylchloride plastics, and recycled thermal paper. BPA metabolites are known to cross the blood-placental barrier (Schönfelder et al., 2002; Sun et al., 2002). BPA has been detected in most of the human body fluids including serum (Takeuchi & Tsutsumi, 2002), urine (Calafat et al., 2008), breast milk (Ye et al., 2006), amniotic fluids (Yamada et al., 2002), ovarian follicular fluid (Ikezuki et al., 2002), and umbilical cord blood (Kuroda et al., 2003). Increasing evidence of cause-and-effect relationship between BPA exposure and female reproductive disorders have been obtained from animal experiments as well as cell culture-based in vitro studies (Palioura & Diamanti-Kandarakis, 2015; Bloom et al., 2016; Ziv-Gal & Flaw, 2016; Nelson et al., 2020; Pivonello et al., 2020). However, the precise biochemical and molecular mechanism(s) by which BPA interferes with steroidogenesis in the ovarian cells still remain unclear. Therefore, the purpose of this study was to discover the steroidogenic biomarker(s) associated with BPA treatment in human granulosa cell line, KGN.
MATERIALS AND METHODS
3,(4,5-dimethylthiazol-2-yl)-2,5-diphenyltetrazolium bromide (MTT), propidium iodide (PI), and Rhodamine 123, and follicle stimulating hormone (FSH) were obtained from Sigma-Aldrich (St. Louis, MO, USA). Complete Protease Inhibitor Cocktail Tablet was from Roche Applied Science (Mannheim, Germany). Dichlorofluorescein diacetate (DCF-DA) fluorescent probe was purchased from Invitrogen (Carlsbad, CA, USA). The western enhanced chemiluminescence (ECL) detection reagent was purchased from Bio-Rad (Hercules, CA, USA). Anti-actin antibody was purchased from Santa Cruz Biotechnology (Santa Cruz, CA, USA). Anti-aromatase antibody was obtained from Acris Antibodies (SM2222P; San Diego, CA, USA). Anti-StAR antibody was purchased from Abcam (ab96637; Cambridge, UK). Anti-rabbit and mouse IgG-conjugated with horseradish peroxidase were purchased from Cell Signaling Technology (Beverly, MA, USA).
KGN cells (purchased from the RIKEN Bioresource Centre, Tsukuba, Japan) were cultured with DMEM/F12 medium to contain 1.2 g/L of NaHCO3, 20 mM HEPES, 50 μg/mL gentamicin, and 10% fetal bovine serum (pH 7.4) at 37°C in a humidified atmosphere with 5% CO2. The cells (5×105 cells) were seeded in gelatin-coated 6-well plate and BPA or FSH was treated at confluence with approximately 50%–60%.
Cells were seeded in 12-well plates at a density of 1.5×104 cells per well (24-well plate). After treatment for 48 h, the culture medium was removed and replaced with a medium containing 0.5 mg/mL of MTT dissolved in phosphate buffered saline (PBS) (pH 7.2). After 4 h, the formed crystals were dissolved in 200 μL of dimethyl sulfoxide (DMSO) and the optical density of the crystals was measured at a wavelength of 490 nm using a microplate reader (BIOTEK EL-312e, Winooski, VT, USA).
The cells (5×105) were incubated with 5 μM Rhodamine 123 dye at room temperature (RT) for 30 min, washed and resuspended with PBS, and then the fluorescence [red (585/590 nm); green (510/527 nm)] was measured with a flow cytometer.
ROS generation was monitored by staining cells with DCF-DA. Following exposure to AsTO, cells were incubated with 5 μM DCF-DA at 37°C for 30 min. The cells were trypsinized, washed with PBS, suspended in PBS, and analyzed using flow cytometry.
For Western blot analysis, the lysed soluble proteins were collected, and the protein concentration was determined with a BCA protein assay kit (Pierce, Woburn, MA, USA). The same amount of protein was subjected to SDS-PAGE and transferred onto a nitrocellulose membrane. The membranes were incubated at RT with a primary antibody in Tris-buffered saline containing 0.05% Tween-20 [TBS-T (pH 7.4)] and 5% nonfat dry milk. Secondary antibody reactions were performed with an appropriate source of antibody conjugated with horseradish peroxidase (HRP). The signals were detected with ECL detection reagent in the LAS-4000 (Fuji, Tokyo, Japan).
Harvested cells were attached to glass slides by cytospin centrifugation. The cells were fixed with 4% PFA, washed with PBS, and incubated with 0.2% Triton X-100. Cells were then incubated with the appropriate primary antibody in 1% bovine serum albumin at RT. For the secondary antibody reaction, cells were incubated with an appropriate fluorescence-conjugated secondary antibody at RT. If required, for counterstaining of the nucleus, the cells were incubated either with PI (50 μg/mL) at RT. Finally, the cells were mounted on glass slides and observed under a Confocal microscope (LSM700, Carl Zeiss, Oberkochen, Germany) at the Neuroscience Translational Research Solution Center (Busan, Korea).
Total RNA was isolated using RNeasy mini kit (Qiagen, Hilden, Germany) and five micrograms of total RNA were used as a template for reverse transcription using a Superscript Reverse Transcriptase kit (Invitrogen). The qRT-PCR was performed by SYBR Green method using the specific primers and SYBR Premix Ex Taq II (Takara, Japan) for StAR and aromatase. The reaction was achieved by Applied Biosystems 7500 Real-time PCR system (Thermo Fisher Scientific, Waltham, MA, USA). StAR: forward (5’-GTCCCACCCTGCCTCTGAAG-3’) and reverse (5’-CATACTCTAAACACGAACCCCACC-3’). Aromatase: forward (5’-GGCATACCTCC TATGGGTTGTC-3’) and reverse (5’-GTAGCCTGGTTCTCTGTGTGAA-3’). Actin: forward (5’-CACTCTTCCAGCCTTCCTTCC-3’) and reverse (5’-AGGTCTTTGCGGATG TCCAC-3’ ). The cDNA was amplified by 40 cycles of denaturation at 95°C for 20 s, annealing at 57°C for 45 s and extension at 72°C for 20 s. During the first cycle, the 95°C step was extended to 5 min. The β-actin gene was amplified in the same reaction as the reference gene. The obtained values were used to calculate the gene/β-actin ratio, with a value of 1.0 used as the control.
Data were expressed as the mean±SD of at least 3 independent experiments. For multiple group comparisons, one-way ANOVA followed by Tukey’s test was performed by GraphPad Prism software (version 4.0; GraphPad, San Diego, CA, USA). Mean values were considered significantly different at p<0.05.
RESULTS AND DISCUSSION
Ultimately, to develop an appropriate cell culture system for high-throughput screening (HTS), it is important to acquire cytotoxicity data before applying the chemicals or compounds of interest. Otherwise, the data obtained from HTS may be inaccurate given that the cells may not be viable or may be dead owing to the toxicity of the tested chemicals. Therefore, we first measured cell viability, mitochondrial membrane potential (MMP), and reactive oxygen species (ROS) levels in KGN cells after exposure to BPA. Thus, we observed that exposure to various concentrations of BPA (0.01, 0.1, 1, and 5 μM) did not significantly reduce the viability of KGN cells following treatment for 48 h (Fig. 1A). This finding is consistent with the results of a previous study (Huang et al., 2020). The number of apoptotic cells (A0; sub-G0/G1 population), determined via flow cytometry, did not also show any increase in cell death compared to that number observed for the control (data not shown). However, both MMP and ROS were slightly increased under 1 μM BPA treatment compared to the control (Fig. 1B and C). The MMP showed signs of hyperpolarization after BPA exposure (Fig. 1B), indicating that the BPA concentrations chosen for this study were adequate for biomarker discovery in KGN cells.
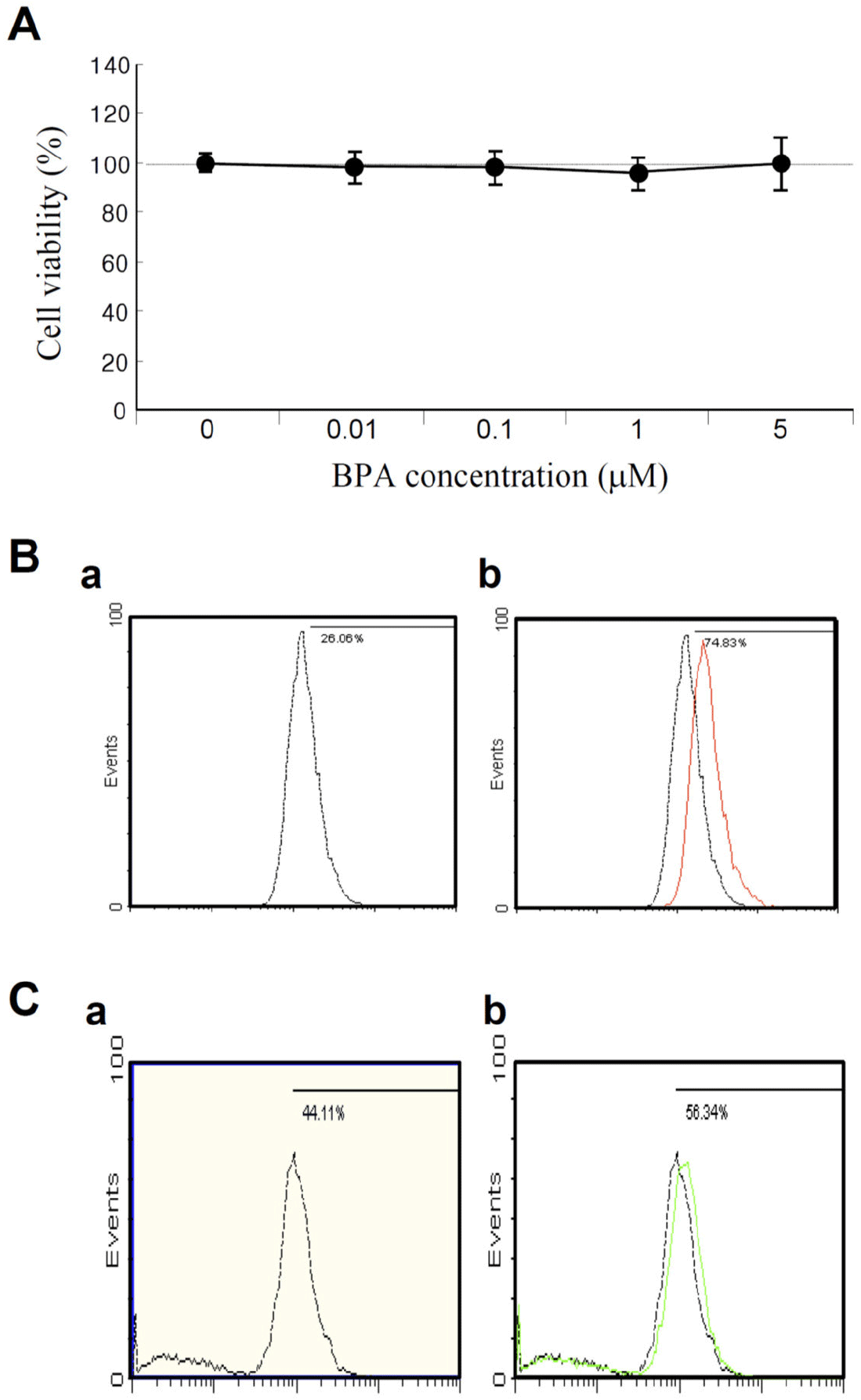
Recently, KGN cells have been used as a cellular target model of female reproduction and endocrinology to test not only cytotoxicity, but also endocrine-disrupting activity (Pierre et al., 2009; Kwintkiewicz et al., 2010; Watanabe et al., 2012; Ernst et al., 2016; Dilaver et al., 2019; Tremblay & Sirard, 2020). Although KGN cells originate from human granulosa-like tumor cells, they not only express aromatase and FSH receptors, but also possess steroidogenic activity; thus, they can secrete pregnenolone and P4 (Nishi et al., 2001). However, they secrete little or no A4 or E2. Our preliminary results (data not shown) obtained via the analysis of steroidogenesis-related protein expression in KGN cells using qPCR and western blot analyses revealed that the expression levels of StAR and aromatase decreased considerably and gradually after BPA treatment in a dose-dependent manner under BPA treatment (Fig. 2A). Further, remarkable decreases in their expression levels at the cellular levels were also confirmed via immunocytochemistry (Fig. 2B), and subsequent StAR and aromatase mRNA expression levels showed profiles similar to those observed for their proteins (Fig. 3), i.e., both StAR and aromatase mRNA expression levels were significantly decreased under BPA treatment at concentrations ≥0.1 μM (Fig. 3). Next, we tested the effect of BPA treatment on FSH-induced StAR and aromatase expression (Fig. 4). Thus, we observed that FSH upregulated StAR and aromatase protein expression levels; however, this effect was suppressed in the presence of BPA (Fig. 4). A similar observation was made in FSH-stimulated KGN cells, but not in StAR (Kwintkiewicz et al., 2010). Furthermore, BPA exposure (5 μM) downregulated aromatase expression induced by dexamethasone and/or oncostatin M (Watanabe et al., 2012). In contrast to our results, a previous study reported the upregulation of StAR gene expression in KGN cells after exposure to BPA (10 μM) (Qi et al., 2020). Further, in this study, a questionable discrepancy was observed: increased StAR expression and decreased P4 synthesis. Another study showed that P4, E2, and the E2/T ratio are significantly decreased after BPA (0.5 and 5 μM) treatment (Shi et al., 2021). A BPA action mechanism study suggested that the β-catenin-FOXL2-CYP191 pathway is associated with E2 production stimulated by a low BPA dose (10 pM) (Liu et al., 2021).
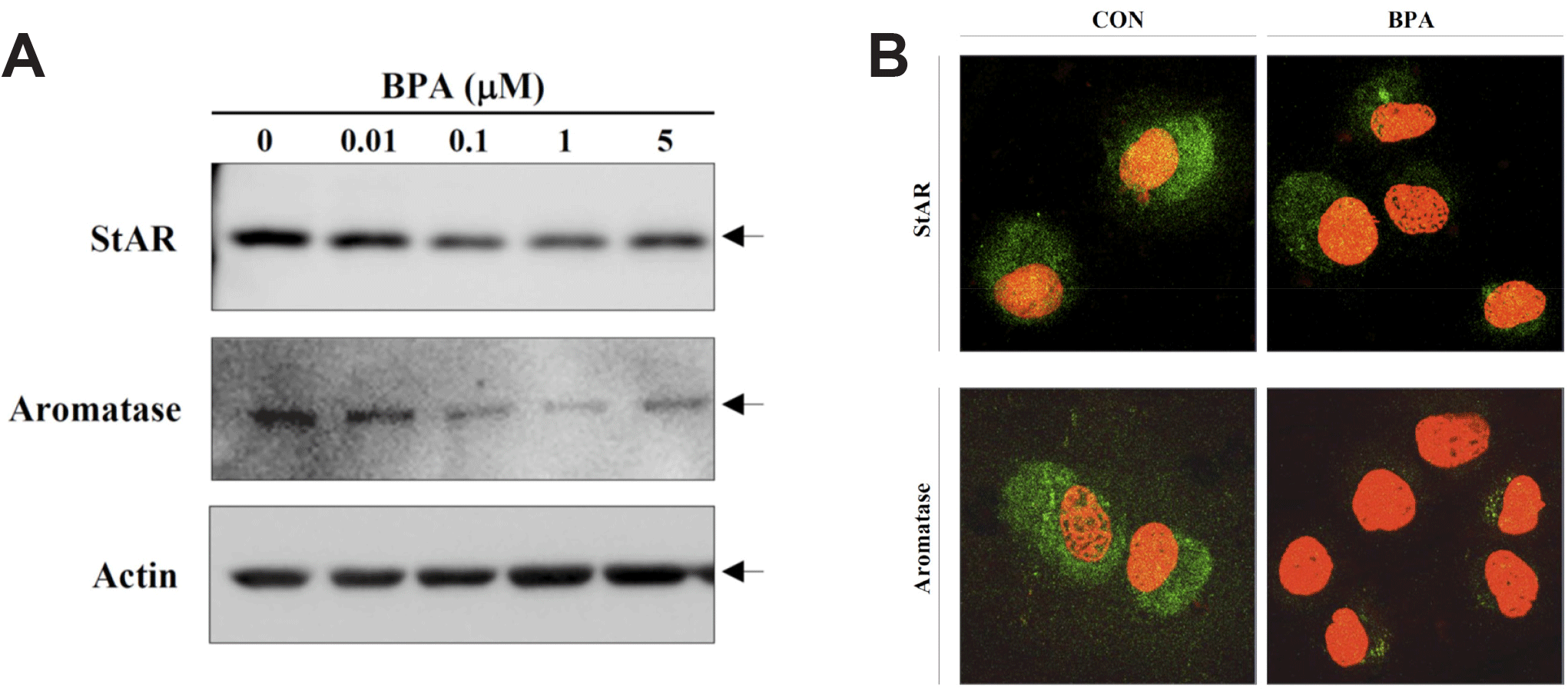
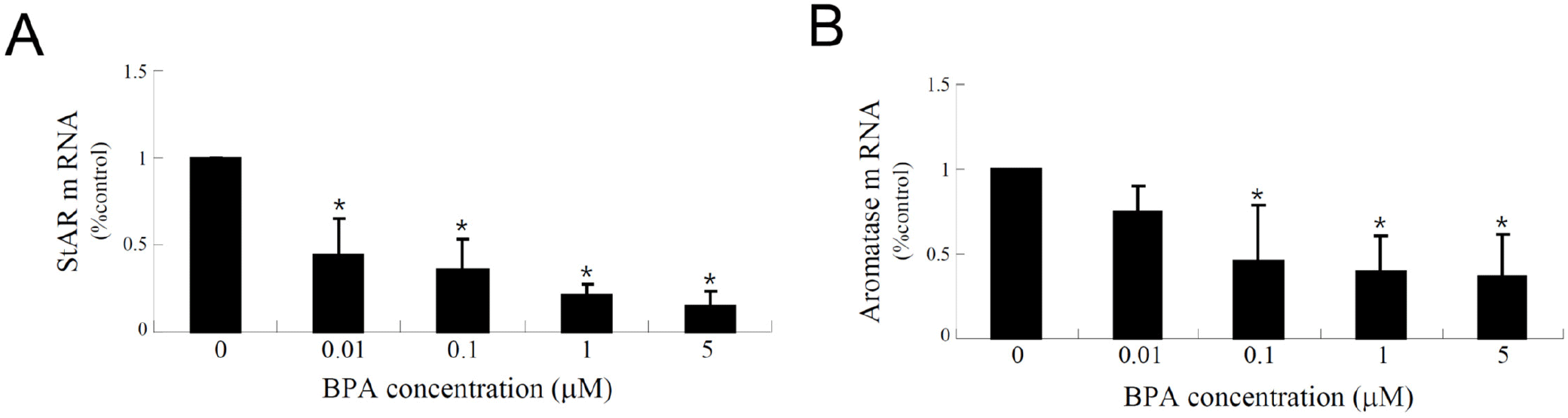
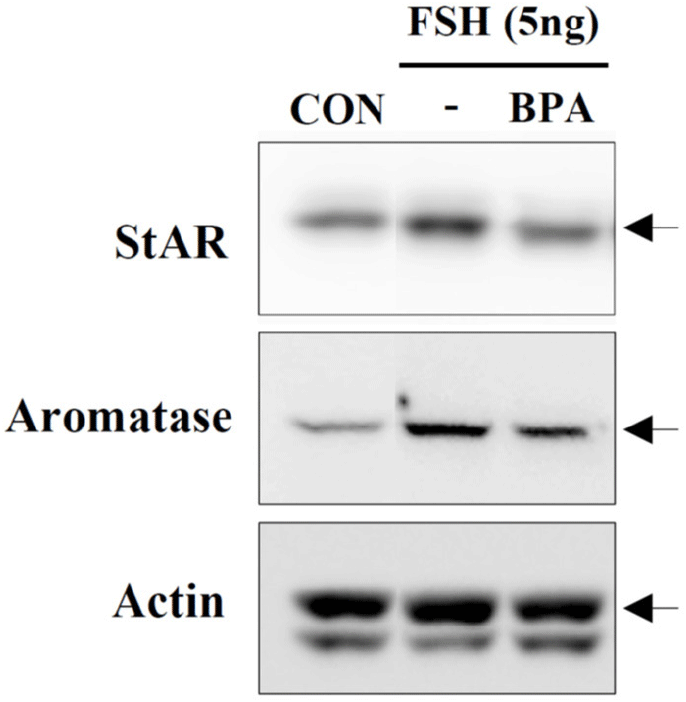
Regarding the steroidogenic effects of BPA on KGN cells, controversies remain regarding the ultimate outcomes. This could be due to several factors, such as the generation of the cell lineage used, cell confluency, and cell culture conditions (type of medium and serum, supplements, etc.). Nevertheless, we believe that the results here presented imply that KGN cells have a good cellular and steroidogenic machinery for evaluating endocrine disruption, even at low doses of environmental or exogenous chemicals. Therefore, StAR and aromatase could be stable and sensitive biomarkers in KGN cells for the cellular screening of the potential risk posed by exogenous and environmental chemicals to female reproductive (endocrine) function.