INTRODUCTION
Perivascular stem cells (PVCs), originating from mesenchymal stem cells (MSCs), have greater proliferation and differentiation potentials compared to those of bone marrow-derived MSCs (Crisan et al., 2008). Among the PVC sources, human umbilical cords (HUC) have some advantages over other tissues because they are easily accessible as a clinical waste product and have low inherent immunogenicity (Nagamura-Inoue et al., 2014). For these reasons, HUCPVCs have drawn considerable interest as a promising material for regenerative medicine. In recent years, the therapeutic effects of HUCPVCs on various diseases have been reported. Tsang et al. (2013) showed that HUCPVCs contributed to skeletal regeneration by generating a matrix and recruiting resident progenitors in a bone defect model. HUCPVCs also contributed to the repair of injured lung tissue as well as protected against neurodegenerative diseases through paracrine effects (Montemurro et al., 2011; Appaix et al., 2014). Thus, the identification and functional assessment of internal and external factors regulating PVC functions are critical for improving their therapeutic potential.
Potassium channels, the largest group of pore proteins, are widely distributed in various cell types (Day et al., 1993; Park et al., 2007). They selectively regulate the flow of potassium (K+) ions across cell membranes (Day et al., 1993). K+ channels have important roles in maintaining normal functions and physiological homeostasis in various cell types as well as regulating the cell cycle, differentiation, and maturation (Kawano et al., 2002). Although several studies have characterized the electrophysiological properties of MSCs and pluripotent stem cells (Heubach et al., 2003; Li et al., 2005; Wang et al. 2005; Bai et al., 2007; Park et al., 2007; Jiang et al., 2010; Park et al., 2013; Tarasov et al., 2017), the expression and function of ion channels in HUCPVCs have not been studied. Therefore, for therapeutic applications, we need to investigate not only the multi-lineage differentiation capacity, but also the electrophysiological properties of K+ channels in HUCPVCs. In the present study, we investigated the expression pa tterns of big-conductance Ca2+-activated (BKCa) and vol tage-dependent K+ (Kv) channels in undifferentiated HUCPVCs and examined the effects of high glucose (HG, 25 mM) on the expression levels of BKCa and Kv channels.
MATERIALS AND METHODS
HUC tissues (n = 3) were obtained from mothers undergoing Caesarian sections, with written informed consent after approval by the Institutional Review Board of Kangwon National University Hospital. The isolation and culturing of HUCPVCs were performed as described previously (An et al., 2015a). Briefly, HUCs were rinsed with sterile phosphate-buffered saline (PBS; Sigma-Aldrich) and incised along their length in dishes. Both ends of the isolated vessels were ligated with black silk and then transferred into 100 mm culture dishes containing α-minimal essential media (MEM) supplemented with 10% fetal bovine serum (FBS; Hyclone), 1% penicillin-streptomycin (Sigma-Aldrich) and amphotericin B (0.3 µg/mL; Sigma-Aldrich). After 7-10 days, the vessels were removed from the dishes and the colonies were subcultured. Once the cells reached 80% confluency, they were passaged by treatment with 0.05% trypsin-EDTA (Sigma-Aldrich). To evaluate the effect of high glucose on the expression of ion channels in PVCs, the cells were plated at a density of 4×105 cells in 35 mm dishes and cultured in α-MEM medium supplemented with low glucose (LG) (1.0 g/L, 5 mM) and HG (4.5 g/L, 25 mM) for 5 days.
HUCPVCs were dissociated into single cells using trypsin-EDTA and resuspended in 1% FBS-PBS. The cells were filtered through a 70 µm cell strainer and reacted with the following antibodies conjugated with fluorochrome for 1 hr at 4℃: CD31-phycoerythrin (PE), CD34-fluorescein-isothiocyanate (FITC), CD45-allophycoerythrin (APC), CD44-APC, CD90-APC, CD146-FITC, and SSEA-4-FITC (all, BD Biosciences). The dead cells were excluded with 7-aminoactinomycin D. Stained cells were analyzed using the FACSCanto II (BD Biosciences) and data were analyzed by FlowJo software (FlowJo).
Total RNA was isolated using the RNeasy Mini Kit (Qiagen) according to the manufacturer’s instructions. Briefly, total RNA was reverse transcribed by using the TOPscript™ RT DryMIX kit (#RT200; Enzynomics). Transcripts were quantitated using TOPreal™ qPCR 2X PreMIX (#RT501S; Enzynomics) and the ABI StepOnePlus™ System Instrument (Applied Biosystems). The expression levels of BKCa and Kv channel genes were normalized to glyceraldehyde 3-phosphate dehydrogenase (GAPDH) and the relative quantification was performed using the comparative CT method according to the manufacturer’s instructions (Applied Biosystems). The primer sequences are listed in Table 1.
RESULTS
We obtained PVCs from the vessels of HUC tissues using a non-enzymatic (NE) isolation method (An et al., 2015b). To prevent cross contamination with hematopoietic and endothelial cells, both ends of the vessel were ligated and then plated into dishes. On days 7-10 post-plating, PVC colonies were subcutured and successfully expanded. HUCPVCs exhibited fibroblast or spindle-like morphology (Fig. 1A). The phenotypic expression of HUCPVCs (Passage 2) was analyzed by flow cytometry. The cells were positive for CD146 (93%), SSEA-4 (10.1 %), CD44 (99.9 %), and CD90 (99%), and negative for CD31, CD45, and CD34, which precluded contamination by endothelial and hematopoietic cells (Fig. 1B). These results suggested that the NE method enabled us to obtain a homogenous PVC population and that the expression pattern of surface markers on HUCPVCs was in agreement with that of human PVCs reported in previous studies.
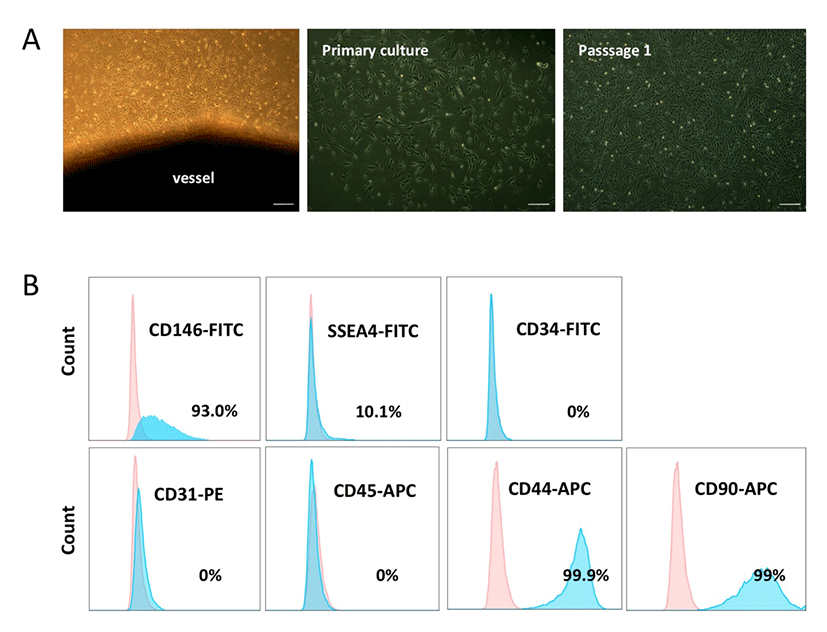
We first investigated the expression patterns of BKCa and Kv channel subtypes in undifferentiated HUCPVCs. We found comparable mRNA expression of KCaβ3a and Kv1.3 subtypes in undifferentiated HUCPVCs (Fig. 2A and 2B). The expression levels of BKCa1.1, BKCa2.2, KCaβ, Kv3.3, and Kv6.1 subtypes were relatively low (Fig. 2A and 2B). High concentrations of glucose in the blood affected the growth and functions of endogenous stem cells. Thus, we next asked if high glucose influenced the expression levels of ion channels. HUCPVCs were plated at a density of 4×105 cells in 35 mm dishes and cultured in both LG and HG conditions for 5 days. HG did not induce morphological changes in HUCPVCs (Fig. 2C), but reduced the proliferative activity (data not shown). HG-treated HUCPVCs showed increased mRNA levels of BKCaβ3a, BKCaβ4, Kv1.3, Kv1.6, and Kv6.1 subtypes. We confirmed the upregulation of BKCaβ4, Kv1.6, and Kv6.1 transcripts in HUCPVCs exposed to HG using quantitative real-time PCR (Fig. 3). These results suggested that BKCa and Kv channels may have unique functions in the growth and differentiation of HUCPVCs, which could be affected by HG exposure.
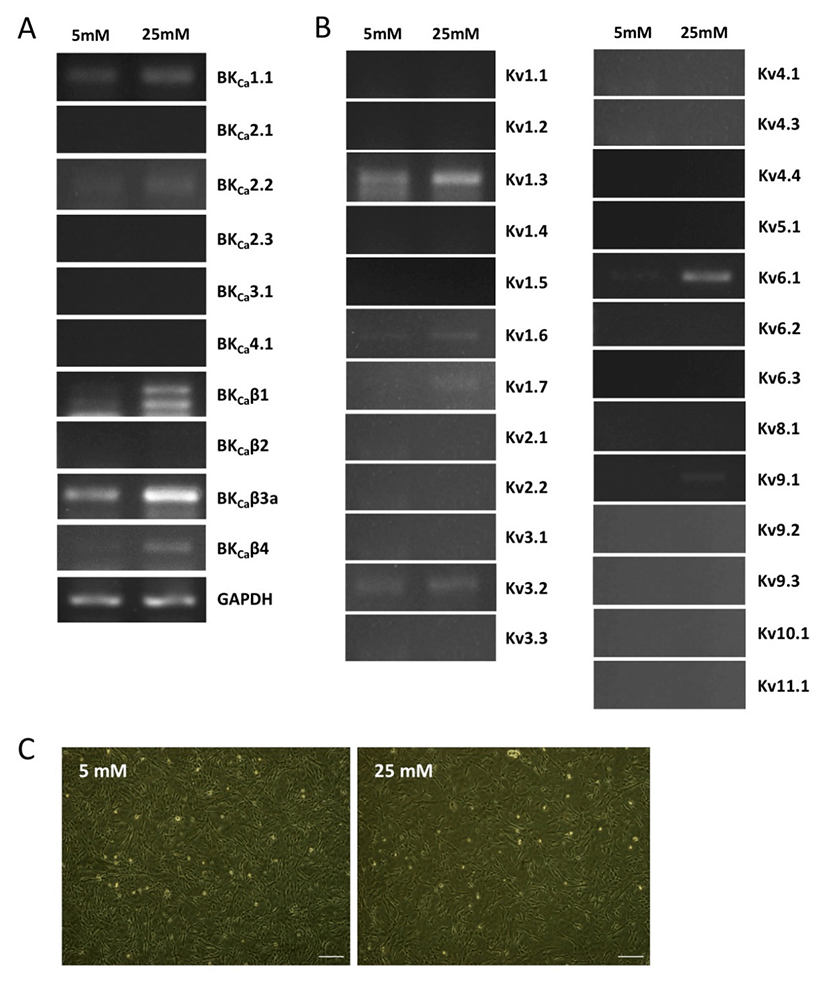
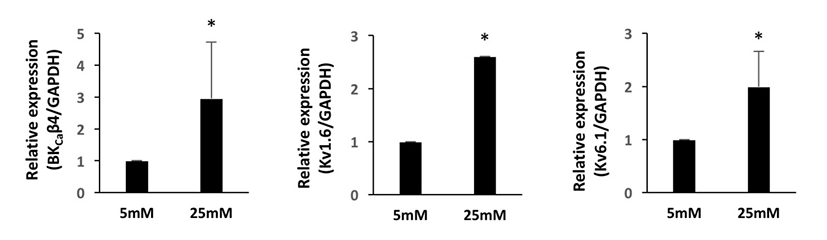
DISCUSSION
In the present study, we showed for the first time the expression patterns of BKCa and Kv ion channels in HUCPVCs, which could be altered by exposure to HG. In recent years, PVCs have been suggested as a promising source for cell-based therapy due to their greater regenerative potential (Crisan et al., 2008). Ion channels are detected in a variety of cell types and play fundamental roles in controlling cell proliferation and differentiation as well as maintaining homeostasis (Kawano et al., 2002). Therefore, we investigated the electrophysiological properties of HUCPVCs to improve their proliferative and regenerative capacities.
Recent studies reported the electrophysiological properties of multipotent MSCs and found that the expression patterns of ion channels were relatively heterogeneous among MSCs. While similar expression levels of Kv1.4, Kv4.1, Kv4.2, and Kv4.3 subtypes were observed between adipose tissues (AD)- and bone marrow (BM)-derived MSCs, expression of Kv1.1, Kv1.4, and Kv7.3 subtypes varied across the tissues or among studies (Heubach et al., 2003; Li et al., 2005; Bai et al., 2007; Park et al., 2007; Park et al., 2013). Bai et al. (2007) could not detect Kv4.1 subtype in AD-MSCs. However, Park et al. (2013) reported strong expression of Kv4.1 subtype in AD-MSCs. The heterogeneity of Kv ion channels in MSCs may be attributed to variations among donors or the tissues from which MSCs are isolated. Our data showed different expression patterns of ion channel subtypes in PVCs compared with AD- and BM-MSCs, indicating that PVCs are different subpopulations and are more homogeneous than MSCs. Culture conditions might also influence the expression of specific ion channel subtypes detected in MSCs. For example, BM-MSCs cultured in HG medium exhibited senescence and genetic instability by upregulation of autophagy and oxidative stress (Chang et al., 2015). In addition, hyperglycemia impaired the proliferation and function of endogenous stem and somatic cells such as MSCs, hematopoietic stem cells and Müller cells (Kim et al., 2015; Kocabas et al., 2015; Manea et al., 2015; Hadarits et al., 2016; Qiu et al., 2016). Thus, we assumed that the glucose concentration in the culture medium may affect the expression levels of ion channels in PVCs and found increased levels of BKCaβ3a, BKCaβ4, Kv1.3, Kv1.6, and Kv6.1 transcripts in HG-treated PVCs compared to those of LG-treated PVCs.
In summary, this is the first characterization of ion channels in HUCPVCs, which provides fundamental information to improve the regenerative capacity of HUCPVCs. Further study will be needed to define the physiological roles of specific ion channels in the proliferation and differentiation of HUCPVCs.