INTRODUCTION
Endocrine disrupting chemical (EDC) is prevalent in the environment (Fox et al., 2004; Choi et al., 2016; Monneret, 2016), and animals can be exposed to EDC via their occuations, ingestion of food (Fox et al., 2004), dust and water (Rudel et al., 2009), and skin (Davey et al., 2007; Kabir et al., 2015). Those EDCs can affect human and wildlife animal reproductive performance (Culty et al., 2008; Phillips et al., 2008; Robins et al., 2011). Based on the dogma of pharmacology, the dose makes the poison (Vandenberg et al., 2012), and most of the studies about EDC have been focused in the toxicological concepts for decades (Swedenborg, 2009; Vandenberg et al., 2012). However, some researches have suggested that EDCs can act in the nanomolar to micromolar range or at picomolar levels like in natural hormones and can damage human and/or animal health. Besides, research is needed to understand whether low-dose responses are lined to adverse effects and mechanisms. Therefore, it becomes more and more important to know the possible effects of endocrine disrupting chemicals (EDCs) in physiological process (Kawaguchi et al., 2015; Mantovani, 2017).
The environmental xenobiotics can get potential to hormonal signaling disruptors by itself or their metabolites (Bonde et al., 2017). So far, the information about metabolized EDCs and specific exposure is insufficient. The degradation products of nonlyphenol ethoxylate (NPE) included 4-nonylphenol (NP), nonylphenol ethoxylate (NPIEO), nonylphenol diethoxylate (NP2EO), nonylphenol triehoxylates (NP1-3EO), nonlyphenoxyacetic acid (NP1EC), and nonylphenolxyacetic acid (NP2EC) (Sheahan et al., 2002; Swartz et al., 2006). Those belong to aklyphenols and are considered to have weak estrogenic activities in vivo and in vitro testing system (Bøgh et al., 2001; Watanabe et al., 2004; Maruya et al., 2012). It is known that NP binds to estrogen receptors and mimics the work of 17β-estradiol, although the affinity of NP for ESR1 has less magnitude than the affinity of 17β-estradiol (Preuss et al., 2006; Bonefeld-Jørgensen et al., 2007; Soares et al., 2008).
EDCs have been known to produce adversely embryonic development, reproductive, neurological and immune effects in animal (Diamanti-Kandarakis et al., 2009). The possible toxicity of EDC depends on the dosage, duration, and the expose of life stage. Maternal exposure of EDCs can be negatively influenced on parents as well as F1 generation (Bøgh et al., 2001). The NP (100-300 mg/kg/day for 30 days; Aly et al., 2012) during perinatal period or adulthood (100 mg/kg; Duan et al., 2017) has a negative effect on spermatogenesis and sperm quality in vivo fertility. Neonatal expose delay testes decent and adult expose affect particularly on spermatogenesis (250 mg/kg, Dejager et al., 1999a; 400 mg/kg, Dejager et al., 1999b; 8 mg/ kg, Odum & Ashby, 2000). It also can give an effect on other organs and functions such as learning and memory (Jie et al., 2013; Kawaguchi et al., 2015). By such experimental results and warning of ECDs, REACH (registration, evaluation, authorization and restriction of chemicals), European chemical agency (ECHA), and Significant New Use Rules (SNURS) has restricted the percentage of NP as 0.1%.
Recently the possible effects of low dose EDCs were continuously suggested. Low-dose NP has effects in both cell and in vivo levels (Melnick et al., 2002; Xu et al., 2017). Yu et al (2011) explored that 100 µg/kg NP can modulate the production of progesterone in rat granulosa cells. 0.5 mg/kg NP slightly impairs special learning performance in male and female rat (Kawaguchi et al., 2015). In this study, we employed the OECD test guideline 443 “the expended one-generation reproductive toxicity study” to evaluate the possible adverse effects of chronic low-dose effects on the body weight, reproductive organ weight, and reproduction.
Materials & Methods
All experimental animal(s) was studied according to the Guide for the Care and Use of Laboratory Animals published by the national Institutes of Health and to the Test Guideline 443 suggested by OECD and under the Experimental Animals Committee of Sungshin University. Animals (CD-1 mice) were maintained under standard condition; temperature (20–24℃), humidity (45–55%), and light (14 hr light/ 10 hr dark) conditions. They were fed free phytoestrogen diet (2018 Teklad global 18% protein rodent diets; ENVIGO, Madison, WI, USA) and water ad libitum using glass bottles. To get F0 generation, the mice fed phytoestrogen free diet were used. Their offspring with normal estrous cycle was chosen as F0. The pubs were weaned at 21 post-natal days.
Nonylphenol (Sigma-Aldrich, Cat # 46018, CAS # 84852- 15-3) was selected as an endocrine disrupting chemical and used at two concentration based on previous report (Kyselova et al., 2003), 50 µg/L and 500 µg/L in drinking water. The chemical stock solution was prepared in purified water and stored at –20℃. Treatment was proceded according on schedule suggested by OECD test guideline (TG) 443. Briefly, paternal generation (F0) were administered in drinking water during 10weeks (pre-mating; 2 weeks, mating; 2 weeks, post mating included pregnancy and lactation; 6 weeks) and then were anatomized for organ sampling (Fig. 1). F1 were administered for experiment of organ sampling during postnatal day 51 (male) and about postnatal day 28 (female, after vagina opening 3 days).
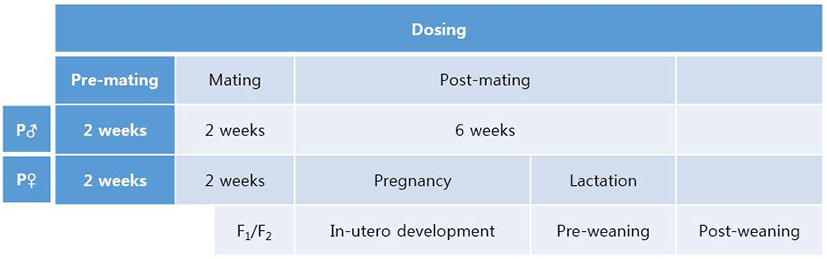
The control and experimental groups of the same sex were sacrificed at the same time. The males and females F0 were sacrificed at end of chemical treatment periods for 10 weeks, and the reproductive organ (testes and epididymis in males; ovary and uterus in females) were excised out
and weighed individually. Relative organs weight was calculated based on organ to body weight. In F1 mice, they were sacrificed at postnatal day 51(male) and postnatal day 28 (female, after vagina opening 3 days) and examined as F0.
To evaluate the number of ovulated oocytes, F0 and F1 generation female (6 weeks old) were superovulated by injection of 2.5 IU pregnant mare serum gonadotropin (PMSG, sigma) followed 48 hr later by injection 2.5 IU of human chronic gonadotropin (hCG, sigma). After 15 hr, the female mice were sacrificed and oocytes were collected from ampulla. The number of sperm was counted with Makler counter chamber (Sefi Medical Instruments LTD, Santa Ana, CA, USA). The caudal sperm collection was followed the method for mouse in vitro fertilization (Hogan et al., 1994).
Result
In male mice, the weights of testis and epididymis were measured as mentioned in Materials and Methods. The weight of testis of F0 male significantly decreased in NP 50 group but not in NP 500 group (Fig. 2). The weight of epididymis significantly increased in NP 50 group but not in NP 500 group (Fig. 2). In F1 male, which were treated from gamete to until sampling, the weights of testis and epididymis significantly decreased and increased in NP 50 groups, respectively (Fig. 2).
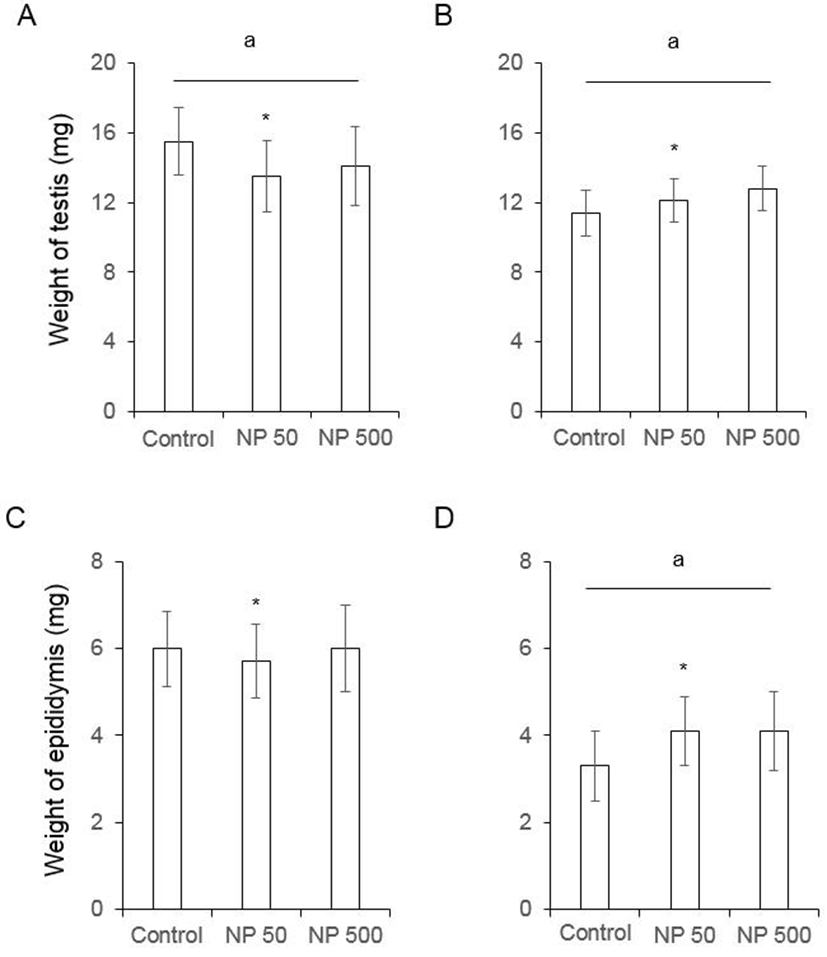
In F0 female mice, that were administered with NP as de picted in Fig. 1, the ovarian weight significantly increased in NP 50 group but not in NP 500 group. However, the weight of ovary was not changed in F1 (Fig. 3). The weight of uterus in F0 female was significantly increased NP 50 and NP 500 groups in concentration-dependent manner. However, in F1 female, which were treated from gamete to sampling (4-5 weeks old), the weight of uterus was not changed (Fig. 3).
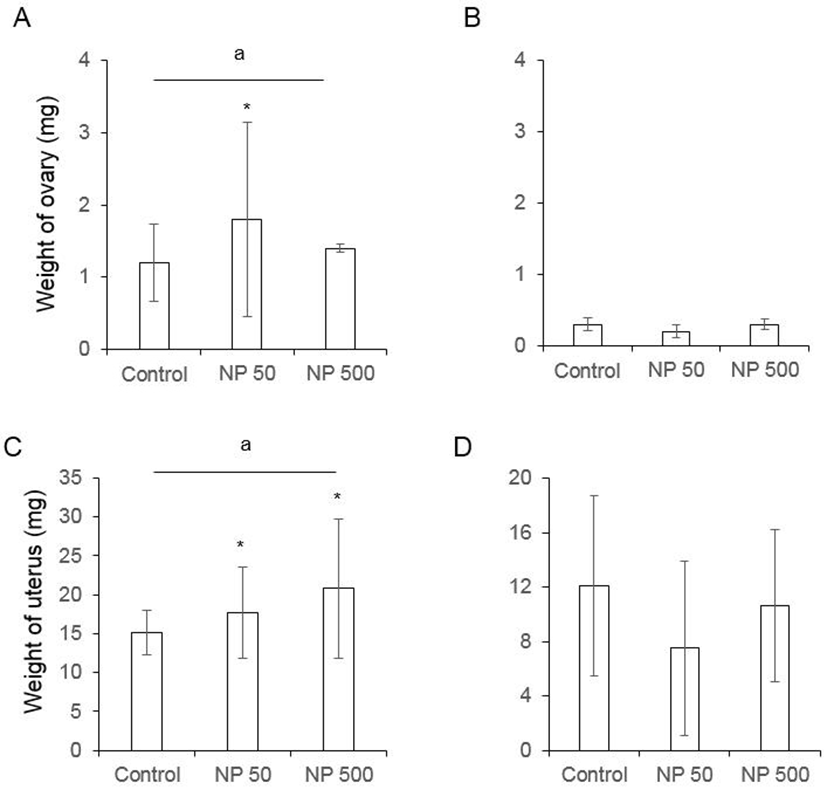
The number of cauda epididymal sperm per body weight was same in controls of F0 and F1 males. Also, in NP 50 and NP 500 groups, it was not changed by generation and groups (Fig. 4). The numbers of ovulated oocytes in F0 were not changed by administration of 50 and 500 µg/L NP (Fig. 5A). In F1 generation, the number of ovulated oocytes was significantly decrease in NP 50 group but not in NP 500 group (Fig. 5B). However, the decrease or increase were not detected in F2 female (data not shown).
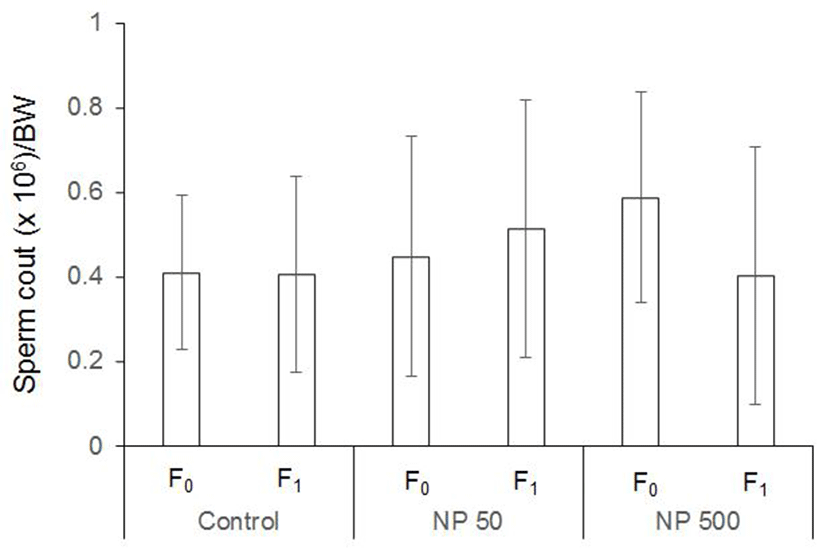
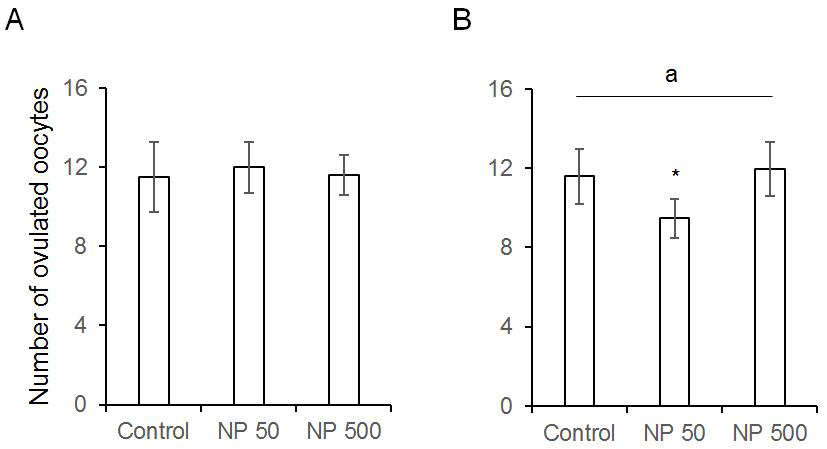
For first 2 weeks we treated low-dose NP, and kept next 2 weeks the males and females in the same cage to mate.
There was no significant difference in litter size in both F1 and F2 generation (Table 1). The sex ratios of pubs were not changed by the expose of NP in all groups of F1 and F2 (Fig. 6). The accumulated mating rate of F0 was not different between groups. The accumulated mating rate was 100% in all groups. The accumulated mating rate was also 100% in all groups of F1 generation (Fig. 7A). In addition, the accumulated rates of successful delivery were not also affected by NP in all groups (Fig. 7B). The gestation length in F0 and F1 was also not changed by the administered NP (Fig. 8).
Generation | Group | No. female | No.delivery | Litter size |
---|---|---|---|---|
F1 | Control | 20 | 20 | 11.5±1.84 |
NP 50 | 20 | 20 | 12.0±3.29 | |
NP 500 | 20 | 20 | 10.8±5.34 | |
F2 | Control | 35 | 35 | 12.0±6.75 |
NP 50 | 40 | 40 | 11.8±6.18 | |
NP 500 | 35 | 35 | 12.0±3.85 |
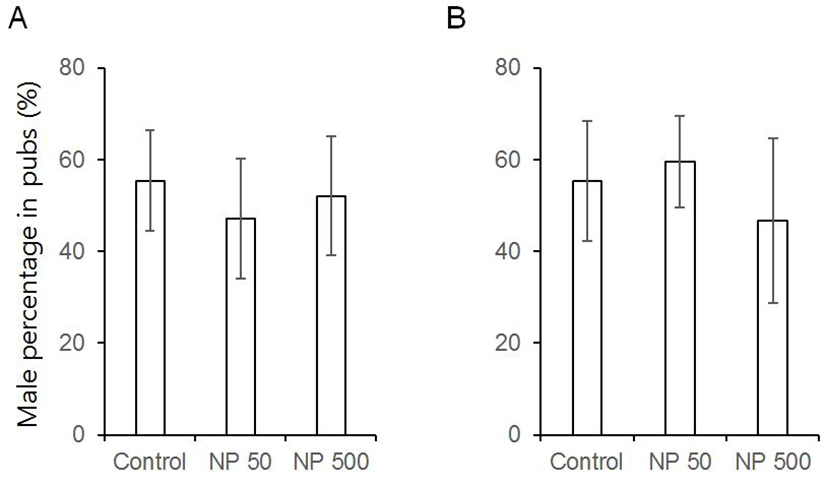
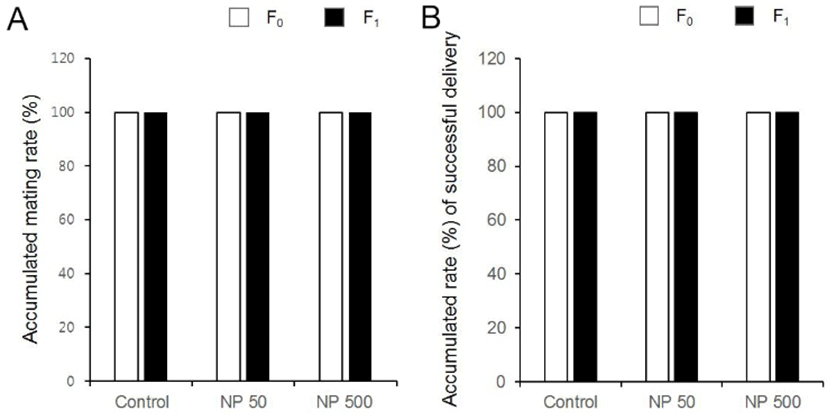
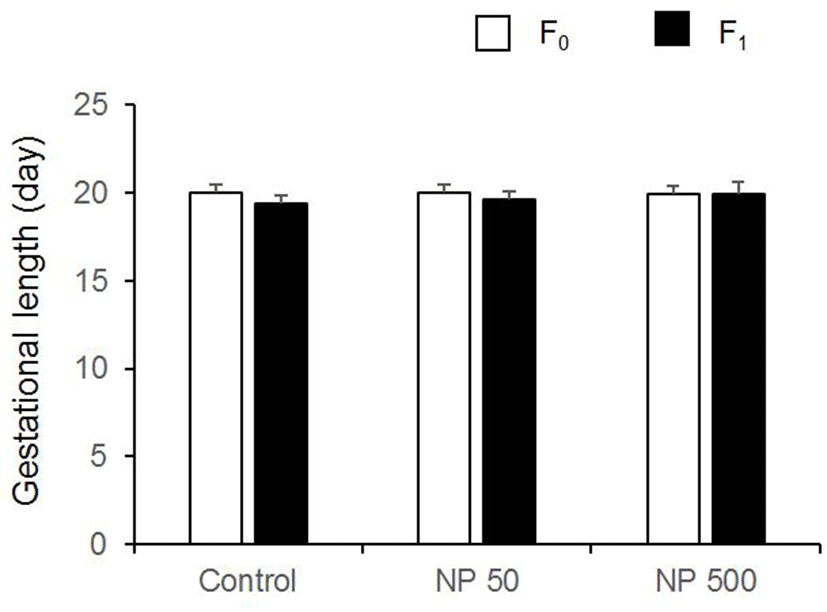
Discussion
Laboratory studies on animals have demonstrated adverse effects of NP, such as on reproduction, development, neurotoxicity and inflammation (Jie et al., 2013; Yücedağ et al., 2015). The responsibility of organisms are various by the exposing dose and duration, and the exposing time of life cycle (Patisaul & Adewale, 2009; Solecki et al., 2017). One of the interesting things is that as anathema to the toxicological principle, the dose response of many hormones and EDCs appears to be nonmonotonic (Patisaul & Adewale, 2009). Lee (1998) suggested that NP (8 mg/ kg/ day, ip) administered from pnd 1 to 10 results in the decreased weight of reproductive organs including epididymis. However, Odum and Ashby (2000) does not conform to the results with Alpk (Wistar derived) rats. Such doseindependent results can be found in gene expression patterns. The genes are expressed in a dose-dependent manner at 0.5-50 µg/kg but not in 50 mg/kg NP (Watanabe et al., 2004). Consistences with those reports, our results show that the effects of NPs did not show dose dependent responsibility. The weight of testis and epididymis decreased or increased on only NP 50 group of F0 and F1, respectively. In ovary, the weight was increased only at 50 μg/L NP in F0.
Estradiol stimulates the proliferation of immature and adult uterine cells and increases the weight (Black and Goode, 1980; Maier et al., 1985). The relative binding affinity of NP to estrogen receptors is 0.035-0.037% and the mean IC50 is 4.00×10–7±0.10×10–7 – 4.40×10–7 ± 0.40×10–7 (Blair et al., 2000). In the uterus, high dose NP (50 mg/kg) has similar effect on most of the genes that are activated by estradiol, and low-dose NP (0.5 and 5 mg/kg) has little effect on the genes that are activated by estradiol (Watanabe et al., 2004). In adults, daily dosing 0.5 and 5 mg/kg NP are equal to 15 and 150 µg NP, respectively. On the other hand, 50 and 500 µg/L NP in daily drinking water (4-7 mL/day/adult) are equivalent to 0.275 and 2.75 µg NP, respectively. Interestingly the uterine weight of parent generation was increased in a concentration-dependent manner. However, in pre-pubertal F1 female, the uterine weights were not different between groups. This means that lower
dose than 15 µg/day can effect on the reproductive organs in a nonmonotonic manner, although NP is 100,000 times less affinity to ERs than estradiol.
Studies show the dimorphic characteristics in males and females to EDCs and suggested female and male germ cells have different susceptibilities to ECD (Anway et al., 2005; Clifton, 2010). Such dimorphism is also supported by our results, as in male mice, the weights of testis and epididymis were affected only by 50 µg/L in both F0 and F1. However in female, the ovary and uterus were affected only in F0. Lower-dose NP did not effect on the weight of ovary or uterus in the pre-pubertal female F1 mice, even though they were exposed from germ cell stage. Parental generation and F1 males treated with 50 and 500 µg/L had similar sperm counts compared with control but not in the number of ovulated oocytes. This means that as expected, the susceptibility to EDC effects of NP changed depending on sex-related endocrine regulation.
It has been suggested that exposing to prenatal and early postnatal EDC might be the causal path of male reproductive disorder such as cryptorchidism, hypospadias, low sperm count and testicular cancer (Bøgh et al., 2001; Rissman & Adli, 2014; Bonde et al., 2017). In addition, altering the epigenic programing of the germ line is observed in the male administered EDCs during fetal gonadal sex determination and such altered epigenic characters are inherited to the subsequent generations (Guerrero-Bosagna & Skinner, 2014). Spermatogenesis in adult male rat that are exposed with NP (100 mg/kg) is affected and the number of sperm decreased (De Jager et al., 1999). However, in meta-analysis, a total of 33 papers provide 89 risk estimates on which there is no strong support for a global effect as a whole or on any specific outcome in male reproduction disorders following prenatal and postnatal exposure (Bonde et al., 2017). Interestingly in this study, the responsibility of testis and epididymis were different between NP 50 and NP 500 groups but the number of offspring, sex ratio of pubs, and accumulated mating rate were not changed. This means that the responding patterns to lower-dose NP in reproduction are different by the character of organs.
Kyselova and colleagues (2003) examined with the same doses except the exposed duration (4 week in CD-1 mice (8 wks old)). Based on the results of Kyselova et al (2003), the detrimental effects on the fertility of sperm of F1 which were exposed to NP according to OECD TG 443 could be suspected. Kyselova et al (2003) evaluated the detrimental effect on acrosomal stability. However, the F1 generation mice had similar responsibility to the NPs in accumulated mating rates and gestational length compared with the control and NP groups. The litter size and sex ratio in F1 and F2 generation were also not different between groups and generations. However, interestingly we did not find any detrimental effects on male fertility. The number of pubs and their sex ratio in F0 and F1 generation were similar between groups and generation. Such difference may be the result of the difference in exposing duration.
The WHO defines the endocrine disruptors as an exogenous substance or mixture that alters the function(s) of the endocrine system and consequently causes adverse effects in an intact organism, or its progeny, or population. The adverse effect refers to “a change in morphology, physiology, growth, reproduction, development or lifespan of an organism which results in impairment of functional capacity or impairment of capacity to compensate for additional stress or increased susceptibility to the harmful effects of other environmental influences” (Solecki et al., 2017). Kyselova et al (2003) suggest that exposing NP (50 and 500 µg/L) to 2 months old male for 4 weeks has multigenerational effect on selective reproductive organ. From our results it is clear that lower-dose NP has characters of EDC in male and female of F0 and F1 generation. However, the chronic exposing of lower-dose NP has no detrimental effect on fertility of the subsequent generation.