INTRODUCTION
In animals, the spermatozoa is the most rapidly moving cell in the body; it fertilizes the ovum and exhibits various species-related morphological differences in the head, midpiece, and tail (Phillips, 1972; Suttle et al., 1988; Morales and Overstreet, 1988; Ward, 1998; Calhim et al., 2007; Bonilla and Xu, 2008; Menkveld et al., 2011). The spermatozoa moves via wave-like movements of the midpiece and tail, and this motility is an obvious marker of survival in a fertile environment. Thus, spermatozoa motility and viability analyses provide data regarding sperm quality and infertility risk, and thus, are important for artificial insemination, in vitro fertilization, and assisted reproduction (Foote et al., 1951; Rikmenspoel, 1965; Smith et al., 1977; Amann, 1989; Vyt et al., 2004).
Sperm motility is currently assessed by visually observing sperms using a microscope. However, this method relies on personal experience and does not present feasible, objective data that could be used by other scientists as a verifiable predictor of the fertilizing potential of spermatozoa (Amann, 1989; Barros et al., 1972; Amelar et al., 1973). Therefore, a computer-assisted semen analyzer (CASA) has been developed to overcome this issue and standardize sperm motility analysis and is considered to be an accurate, rapid, reliable, and objective semen analysis method (Farrel et al., 1998; Coetzee et al., 1999; Amann and Wabersk, 2014). Using CASA, sperm velocity and motility patterns can be accurately analyzed, and automatic assessments of various types of abnormal sperm motility, concentrations, and morphological parameters can be performed. CASA has facilitated the quantification and qualification of various characteristics of semen from many types of animals such as cattle, pigs, poultry, rabbits, dogs, cats, goats, sheep, and humans (Fetterlof and Rogers, 1990; Feldman and Nelson, 1996; Holt et al., 1997; Vyt et al., 2004; Lavara et al., 2005; Betancourt et al., 2006; David et al., 2015). However, despite its usefulness, CASA is expensive and requires an accurate microscope that can distinguish clear contours to identify the sperm head as the center of movement. In this study, we analyzed confocal microscope photos of spermatozoa to develop a simple method of evaluating sperm motility, which is similar to CASA.
Confocal microscopy, frequently called confocal laser scanning microscopy (CLSM), is an imaging technique used for obtaining high optical resolution and contrast via a spatial pinhole placed at the confocal plane of the lens, which eliminates out-of-focus light at specific wavelengths (Patel and McGhee, 2007). CLSM can also generate transmission images by reflecting laser light against an objective sample and converting light photon density into image dot intensity via a digital signal in the targeted area. Image acquisition is based on the principle of scanning; thus, a time difference between the start and end signals of a single image should be manifested. As a result, CLSM images of rapidly moving sperms contain wave-like distortions and bent flagella (including the midpieces and tails). With these data, we realized that CLSM could be used to obtain data regarding sperm motility. In this study, we used simple sperm images obtained using CLSM, rather than the sperm trajectory motility method, to analyze sperm motility and demonstrated flagellar speeds and head movement angles using the real-time mode of CLSM.
MATERIALS AND METHODS
The protocol and standard operating procedures for the treatment of pigs used in this study were reviewed and approved by the Institutional Animal Care and Use Committee of the National Institute of Animal Science, RDA (Approval number: NIAS2014-489, D-grade).
Fresh semen was collected from Landrace-strain boars aged 15-36 months using the gloved-hand method. Five sperm samples were subjected to motility testing after dilution in Beltsvile thawing solution (BTS). Four volumes of BTS were slowly added to 1 volume of semen over a 3-5 min period to reduce dilution shock. Diluted samples were then stored in a low-temperature incubator (17℃).
The cell adhesive CELL-TAKTM (Becton Dickinson, Franklin Lakes, NJ, USA) was used to fix the sperm heads to the culture dishes as follows. A small volume of adhesive (2-3 μL) was applied to the cover glass bottom of confocal dish (SPL Life Science, Gyeonggi-do, Korea). The concentrations of semen dilutions were adjusted to 5-6 × 106 cells/ml with BTS, and approximately 50-100 μL of each adjusted sample was added to a prepared confocal dish and covered with mineral oil (Sigma, St. Louis, MO, USA). The dish was incubated for 5 min at 38.5℃ in a 5% CO2 incubator, following which unbound sperms were removed by washing with BTS. Finally, sequential images of sperm movement were obtained using a confocal microscope in the real-time mode.
Five to ten real-time images of live sperm were scanned at 5-s intervals using a confocal microscope with a 40× objective lens (LSM510, Carl Zeiss, Oberkochen, Germany; FluoView 400, Olympus Corporation, Tokyo, Japan) and one scanning number was used for each images. The Y-axis lengths of the total image and flagellar area were measured according to the scanning times required for image reconstruction using an image analysis program. The flagella imaging time (x, s) could be estimated using the proportional expression of a (µm): b (µm) = c (s): x (s) (Fig. 1A and Table 1). Thus, the flagellar beat speed (N, number/s = n × 1/x) and the number of flagellar movements per second could be calculated. To measure the width of the flagellar movement angle in the left-right direction (Fig. 1B), the angles (d) between the extension lines of a′ and c′ and the extension lines of b′ and d′ were measured.
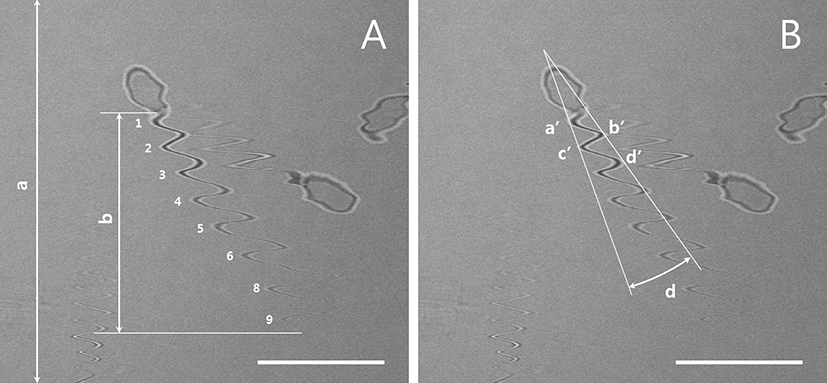
Sperm I.D | Number of flagella motility in image (n) | The length of Y axis in sperm movement (b, µm) | Movement of sperm tail(N, number/sec) |
---|---|---|---|
1 | 9 | 34.8 | 14.25 |
2 | 14 | 38.6 | 20.01 |
3 | 3 | 40.2 | 4.12 |
The measurement of the total Y lengths of the image (a) and sperm tail (b) are illustrated in Fig. 1A. The flagellar activity of the sperm cell with I.D. 1 was recalculated and shown to have normal activity. Representative results from fast-moving (I.D. 2) and slow-moving (I.D. 3) sperms are also shown. I.D., identification number.
RESULTS
The use of real-time CLSM images of living sperm facilitated estimations of sperm speed from the tail trajectory (i.e., wave like) movements. As shown in Fig. 1A, the representative sperm exhibited nine flagellar strokes, leading to a recalculated sperm movement of 14.25 strokes/s; this is shown in Table 1 as an identification number (I.D.) 1 sperm. An analysis of other individual sperms revealed sperm movement ranged from approximately 4 strokes/s (slow moving) to 20 strokes/s (fast moving).
The speeds of fresh and low-temperature-preserved spermatozoa were determined (Fig. 1A and Fig. 2). When porcine semen was preserved at 17℃ for 24 h, the flagellar speed significantly decreased from 11.0±2.3 strokes/s (fresh) to 5.7±1.8 strokes/s (p<0.05).
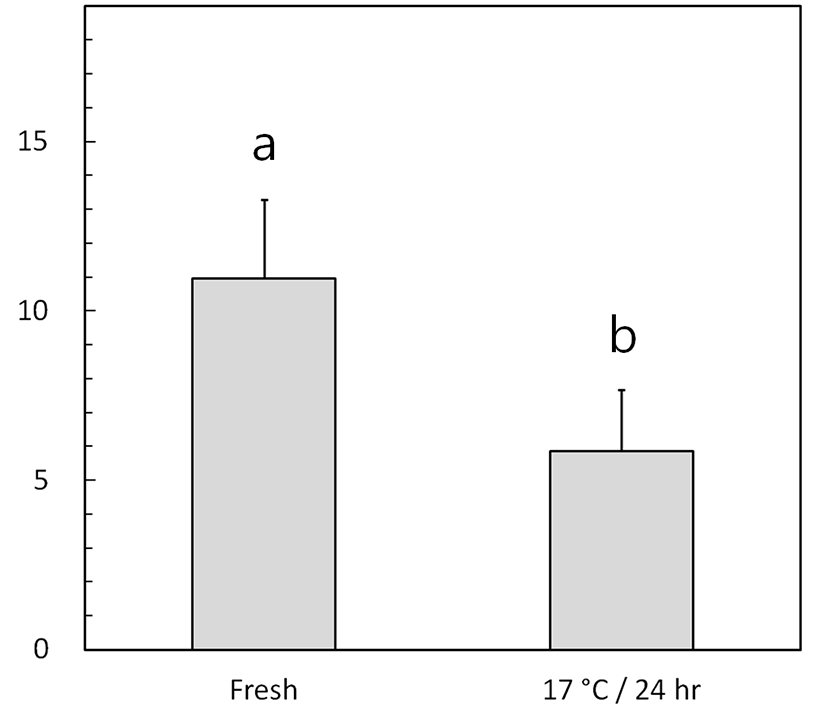
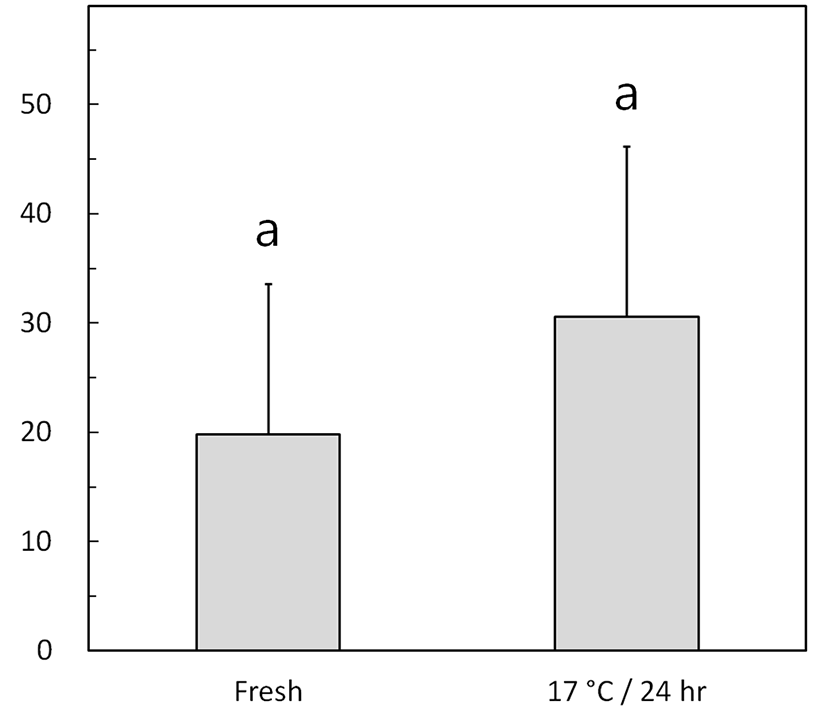
Fig. 3 shows the left-right flagellar movement angles of fresh and low-temperature-preserved spermatozoa using the method described for Fig. 1B. The preserved semen exhibited a nonsignificant increase in the flagellar movement angle, from 19.8°±13.8° (fresh) to 30.6°±15.6° (p> 0.05).
DISCUSSION
In this study, we proposed a simple sperm motility analysis method based on flagellar (i.e., sperm tail) speed and movement angle. As mammalian sperms can regulate motility and migrate to the locus of fertilization in the female reproductive tract (Eisenbach, 1999; Yoshida and Yashida, 2011), an understanding of individual sperm movement is essential for a sperm quality assessment. Although sperm cells were attached to culture dishes with a cell adhesive, the basic rules of sperm movement would be applied for observing free-moving sperms under a low-magnification objective lens. Conventional semen analysis methods such as CASA track sperm movement by distance or trajectory velocity and the speed of sperm head movement per unit time, thereby automatically calculating the number of spermatozoa in a given field (Amelar et al., 1973; Amann and Wabersk, 2014). Because semen samples contain cells with different morphologies and motility patterns, CASA uses a lower magnitude objective lens (10× or 20×) to observe large numbers of spermatozoa. For real-time analyses, sperms should be treated with stimulants or antagonists to simultaneously yield different data from specific spermatozoa. According to the proposed method, CLSM could be used to image moving sperms; therefore, motility data for individual spermatozoa could be easily obtained by measuring other indicators such as calcium or reactive oxygen species.
Fixation of the sperm heads to the bottoms of culture dishes, as described above, could simulate the event of fertilization, thus demonstrating flagellar movement angles. This technique provided data regarding the distinct behaviors of flagella and the directional movements of cells in contact with a surface. In CASA, sperm tail movement can be expressed as a beat cross frequency (BCF; in Hz); except for the lack of attachment, this measurement is similar to our method tested in this study. BCF, which is considered the most constant animal sperm CASA measurement variable, could be used to identify active spermatozoa. Therefore, obtaining data regarding the movements of individual spermatic flagella can facilitate an understanding of sperm physiology by providing a means for experiments regarding individual sperm movement regulation.
This study confirmed that low-temperature preservation reduced sperm motility and affected flagellar activity. Sperm motility is considered an asymmetrical operation that establishes the directionality of movement, a phenomenon that can be easily observed in confocal microscopic images of changes in flagellar movement angles from this and other studies (Rikmenspoel, 1965; Brokaw et al., 1974). In addition, the initial sperm wave motion begins in the neck area and subsequently moves to the tail, and the movement angle determines the directionality of the sperm toward the locus of fertilization by controlling the flagella. We further showed that CLSM imaging of fixed-head spermatozoa could be used for determining the intensity of flagellar movement angles in the left-right direction. The sperm tail movement continuously changes direction to communicate with the ovum via chemotaxis before contact; therefore, flagellar movement angle is an important factor with regard to the mechanism of directionality.
In conclusion, we reported the first simple CLSM-based analysis of sperm movement and directionality. We believe that a related automated analyzing program would accelerate sperm analysis processes.