INTRODUCTION
The uterus secretes various growth factors, cytokines, and nutrients essential for embryo growth, implantation, and gestation. During the estrous cycle, it undergoes morphological and physiological changes such as increased thickness of the glandular epithelium and endometrium, angiogenesis, gene expression, and cytokine secretion (Stroband et al., 1986; Baker et al., 1998; Demir et al., 2010; Franczak et al., 2013). In particular, tissue remodeling of the mammalian uterus, which includes angiogenesis and increased number of secretory cells in the endometrium, provides a suitable environment for the survival and growth of the embryo and implantation of the conceptus. These changes within the uterine microenvironment are regulated by gonadotropins, hormones, and cytokines.
Estrogen is one of the main sex hormones of the female reproductive system and plays an important role in reproductive processes, such as uterine tissue remodeling and implantation recognition (Bazer & Johnson, 2014). This hormone is secreted from the ovaries and affects the endometrium blood vessels. In particular, the porcine conceptus produces 17β-estradiol (E2), a type of estrogen that prepares the endometrium for implantation during the pre-implantation period (Bazer & Johnson, 2014).
Human chorionic gonadotropin (hCG) has the same receptor and similar function as luteinizing hormone (LH) (Bolzan et al., 2013). Generally, it has been used for the induction of estrous and ovulation in pigs (Wongkaweewit et al., 2013) and cows (Giordano et al., 2012). In the mammalian endometrium, prostaglandin (PG) synthesis is regulated by LH via increased PG-endoperoxide synthase 2 in uterine cells, and the uterine environment is altered to begin the luteal phase (Sugino, 2014).
Interleukin-1β (IL-1β) from the conceptus is an important cytokine for implantation in pigs and plays a role in implantation and gestation as an autocrine and/or paracrine factor (Bazer & Johnson, 2014). As a paracrine factor, IL-1β acts on luminal epithelial cells through its receptors (interleukin-1 receptor, IL-1R) (Subramaniam et al., 2004). Thus, understanding the effects of hormones and cytokines on endometrial cells is highly important.
Plasminogen activators (PAs) are serine proteases that are present in two forms-urokinase-type (uPA) and tissue-type (tPA)-in most extracellular fluids, including seminal plasma (Kobayashi et al., 1992), uterine and oviductal fluid (Finlay et al., 1983; Kouba et al., 2000), and ovarian fluid (Beers, 1975). The plasmin converted from plasminogen by the PAs directly or indirectly degrades the extracellular matrix (ECM). Because of this feature, the PA system is associated with physiological processes including angiogenesis (Olofsson et al., 1998), activation of growth factors in the ECM (Menshikov et al., 2006), cell migration (Ploplis et al., 1998), and tissue remodeling (Martin and Arias, 1982). In addition, the PA system regulates the reproductive process. The two types of PAs are released from mammalian cumulus-oocyte complexes and are related to oocyte maturation and fertilization (Ebisch et al., 2008); moreover, plasma and acrosomal membranes of spermatozoa contain PAs to help penetrate the zona pellucida (Sa et al., 2010). In addition, epithelial cells in the oviducts and uterus express PAs and their inhibitors during the estrous cycle (Ahn et al., 2009; Hwangbo et al., 2013). Despite the PA system being closely associated with reproductive processes in the mammalian uterus, mechanisms underlying the regulation and activation of PAs in the uterus of domestic animals remain unclear. Therefore, the present study aimed to investigate changes in the activity and mRNA expression of PAs induced by E2, hCG, and IL-1β in porcine endometrial cells.
MATERIALS AND METHODS
A porcine uterus was collected from a local slaughterhouse and transported to the laboratory within 2 h on ice. The uterus was washed using Hank's Balanced Salt Solution (HBSS) and the ovaries and mesometrium were removed. The inside of the uterus was flushed using HBSS. The uterine horns were then cut along the vertical axis and epithelial cells were collected in Dulbecco's Modified Eagle's Medium (DMEM, Invitrogen, MA, USA) containing collagenase type I (66 unit/mL) by gently scraping the endometrial epithelium using a surgical blade. Epithelial cells were incubated in a shaking incubator (38℃, 120 rpm) to isolate them from the tissues. Isolated cells were filtered using a cell strainer (SPL Life Sciences, Korea) and centrifuged at 1,200 g for 5 min. Blood components were removed using Tris-NH4 and washed in HBSS. Collected cells were cultured in DMEM/F-12 containing 10% (v/v) FBS (Invitrogen), 0.2% (v/v) amphotericin B (Sigma-Aldrich, St. Louis, MO, USA), and 0.5% (v/v) antibiotic-antimycotic (ABAM, Invitrogen) at 38.5℃ in a 5% CO2 incubator, and the culture medium was changed every 48 h. When cells reached 80% confluence, different concentrations of E2 (0.2, 2, 20, and 200 ng/mL), IL-1β (0.1, 1, 10, and 100 ng/mL), or hCG (0.5, 1, 1.5 and 2 IU/mL) were added for 24 h.
For mRNA extraction from cells, samples were treated with RNAiso Plus (Takara, Japan) and rotated for 10 min. Chloroform was then added to the RNAiso Plus followed by vortexing for 5 min. Extracted mRNA was separated by centrifugation (12,000 g, 4℃, 5 min) and washed with isopropyl alcohol followed by 75% ethanol. The dried mRNA pellet was mixed with DEPC-treated de-ionized water and mRNA concentrations were determined using the NanoDrop 2000 (Thermo Scientfic Nanodrop, Wilmington, DE, USA). cDNA was synthesized using Maxime RT PreMix (Intron Biotechnology, Korea) and PCR was conducted using primers (Table 1). The identities of PCR products were confirmed by 2% agarose gel electrophoresis containing ethidium bromide (EtBr, Bioneer, Korea). The relative mRNA expressions of uPA and tPA were normalized to GAPDH (glyceraldehyde 3-phosphate dehydrogenase), and ImageJ was used for image analysis.
uPA, urokinase-type plasminogen activator; tPA, tissue-type plasminogen activator; GAPDH, glyceraldehyde 3-phosphate dehydrogenase.
Samples of the collected culture medium (20 µL) were dispensed into a 96-well microplate and mixed with 30 µL of a plasminogen working solution (Sigma-Aldrich). The solution was incubated at 38℃ for 1 h. After incubation, substrate buffer [0.18 mM Z-L-Lys-SBzl hydrochloride, 0.22 mM 5,5′-dithiobis-(2-nitrobenzoic acid), and 0.01% Triton X-100] was added and was further incubated at 38℃ for 1 h. PA activity was determined by absorbance at the wavelength of 405 nm using a microplate reader.
All numerical data representing each parameter were analyzed using Statistical Analysis System software (SAS, version 9.4). Data are represented as the means±standard error of the mean (SEM) and were analyzed using Duncan's multiple range test. Comparisons among treatment groups were conducted using a generalized linear model in the SAS package. A value of p<0.05 was considered statistically significant.
RESULTS
Although the mRNA expression of uPA and tPA increased in E2-treated uterine cells, this was not significant (Fig. 1). Interestingly, treatment with 0.1 ng/mL IL-1β significantly reduced the mRNA expression of uPA (Fig. 2A, p<0.05), whereas that of tPA remained unchanged. Similar to the latter finding, the mRNA expression of neither PA was altered by hCG (Fig. 3).
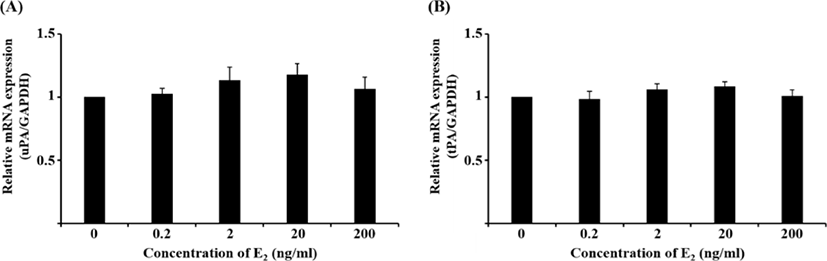
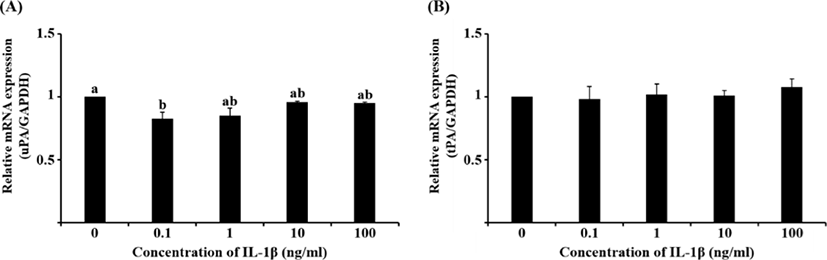
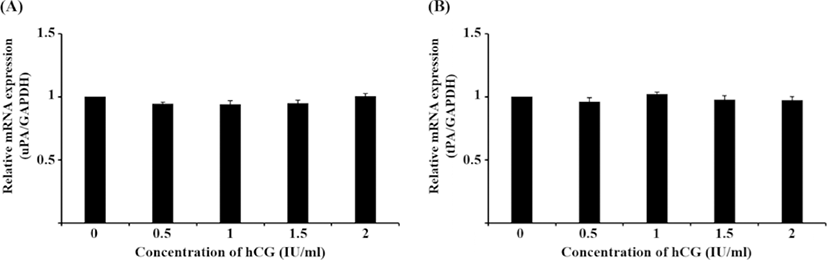
PA activity significantly increased following treatment with 2, 20, and 200 ng/mL E2 compared with the control group (Fig. 4A, p<0.05), which was similar to the tendency in the mRNA expression of PAs to increase. Treatment with 0.1 and 1 ng/mL IL-1β significantly increased PA activity compared with the other groups, whereas 10 and 100 ng/mL IL-1β decreased PA activity (Fig. 4B, p<0.05). This pattern of PA activity was contrary to the mRNA expression of uPA seen following IL-1β treatment. Unlike mRNA expression, PA activity increased in 2 IU/mL hCG-treated cells compared with the other treatment groups; however, none of the hCG groups were significantly different from the control group (Fig. 4C).
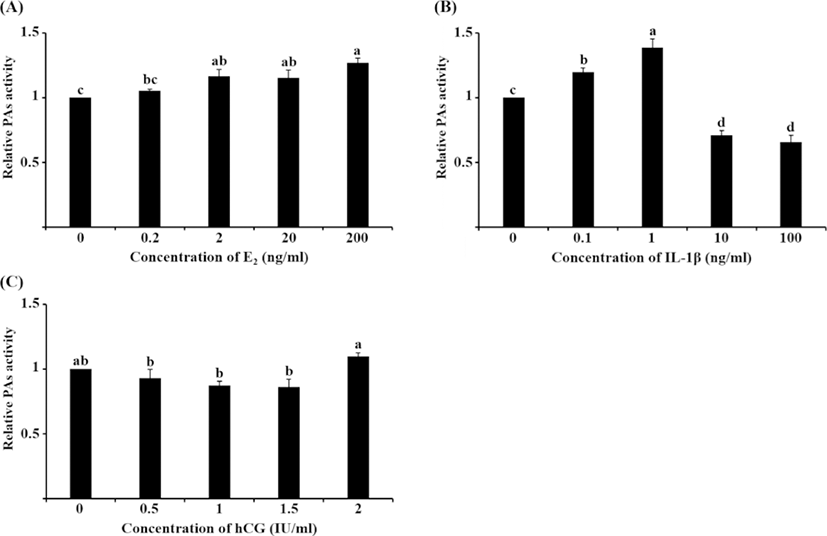
DISCUSSION
This study was conducted to confirm the effects of E2, hCG, or IL-1β on the mRNA expression and activity of two types of PA in porcine endometrial epithelial cells. mRNA expression of uPA decreased only with 0.1 ng/mL IL-1β treatment, whereas that of tPA remained unchanged. Interestingly, altered PA activity resulting from treatment with different concentrations of E2 showed a similar pattern to mRNA expression of PA. Conversely, a pattern of PA activity that was different from that of the mRNA expression of uPA was seen after IL-1β treatment.
During the estrous cycle in mammals, morphological and physiological features of the uterus are altered by a number of hormones, cytokines, and chemokines, and these are important for the survival of the embryo, implantation, and successful pregnancy. Cheon (2007) reported that collagens in the early-pregnant mouse uterus are regulated for successful implantation and pregnancy. In addition, the expression of various genes and proteins that act as essential regulators of uterine function and its microenvironment is controlled through the actions of hormones and cytokines during the estrous cycle. As tissue remodeling factors, PAs are present in the uterine tissue and their levels are altered during the estrous cycle (Kim et al., 2011). They play a role in reproductive and physiological processes including angiogenesis, oocyte maturation, embryo development, ovulation, activation of matrix metalloproteinases (MMPs), and degradation of collagens and ECM proteins (Ebisch et al., 2008). In particular, sperm-zona pellucida binding is decreased by both PAs in the cytoplasm and zona pellucida of porcine oocytes during fertilization (Coy et al., 2012), and Krania et al. (2015) reported that addition of tPA during the in vitro fertilization of bovine oocytes decreased embryo development and increased the expression of apoptosis-related genes in embryos. Therefore, regulatory mechanisms underlying PA mRNA expression and activation in the female reproductive tract are important for animal reproduction.
Steroid hormones, including estrogen, progesterone, and androgens, play a pivotal role in uterine endometrial function in mammals (Cheon et al., 2009). During endometrial growth in the rat uterus, the thickness of the luminal epithelium increased with estrogen treatment (Lai et al., 2000). Estrogen and progesterone regulate reproduction via genomic and non-genomic actions (Stormshak & Bishop, 2008). In the present study, treatment with E2 enhanced PA activity, but did not influence the mRNA expression of PA. This suggests that estrogen may has influenced to translation or post-translation processes for the activation of PAs via non-genomic actions. Furthermore, we expect that an E2-induced increase in PA activity could be responsible for the tissue remodeling associated with angiogenesis, increased growth of the glandular epithelium, and enhanced thickness of the endometrium during the estrous cycle and implantation in pigs. However, effects of estrogen on the translational and post-translational processes of PAs in porcine endometrial cells require further research.
Generally, hCG has been used to induce ovulation in pigs (Brussow et al., 2009). As hCG has a similar structure to LH, it interacts with the LH receptor within the ovary. During the estrous cycle in pigs, a rapid increase in LH concentration known as the LH surge occurs before ovulation, inducing ovulation through rupture of the ovarian wall. At the same time, both uPA and tPA are produced in the granulosa and thecal cells of rat follicles by gonadotropins (Ny et al., 1985; Liu et al., 1987). Kim et al. (2011) reported that PA activity in porcine uterine tissue increased during the post-ovulatory period compared with the pre-ovulatory period. In this study, the activation of PAs in endometrial cells was stimulated by 2 IU/mL hCG treatment. In the uterus and oviducts, hCG influences PG synthase expression and PG synthesis (Shemesh et al., 2001; Malysz-Cymborska et al., 2013), and the actions of hCG in the female reproductive tract are important for gamete transport and survival. PA activity stimulated by hCG may play a role in uterine tissue remodeling and regulation of the intrauterine environment, thereby enabling embryo survival.
During the pre-implantation period, the conceptus trophectoderm of the pig secretes IL-1β, which is associated with elongation and pregnancy recognition (Ross et al., 2003). Interaction between the endometrium and conceptus via IL-1β is an important phenomenon for successful implantation in pigs. In addition, proliferation of uterine epithelial cells is stimulated by IL-1β via activation of the extracellular signal-regulated kinase (ERK) 1/2 mitogen-activated protein kinase (MAPK) signaling cascade (Jeong et al., 2016). In the present study, the mRNA expression of uPA was reduced by a low concentration of IL-1β, al-though tPA mRNA was not affected. PA activity increased in the low concentration groups (0.1 and 1 ng/mL), whereas it decreased by 10 and 100 ng/mL IL-1β. ERK/MAPK signaling regulates cellular processes including gene expression, protein synthesis, cell migration, and proliferation. The activation of IL-1β-induced ERK/MAPK signaling has been reported to be dose- and time-dependent (Jeong et al., 2016). Ny et al (1985) reported that the two types of PAs were differently regulated in granulosa cells by gonadotropins. Therefore, differential patterns of the mRNA expression of PA and PA activity may be regulated by the IL-1β-mediated ERK/MAPK signaling cascade.
In this work, we found that the mRNA expression of uPA and tPA is differently regulated by IL-1β in porcine endometrial cells, and treatment with E2, hCG, or IL-1β influenced the activation of PAs. During the estrous cycle and implantation period, the PA system plays an important role in tissue remodeling, including angiogenesis and effects on secretory glands and thickness of the endometrium. These results suggest that regulation of PA expression and activation by hormones and cytokines in the porcine uterus is critical for successful pregnancy. Understanding the PA regulatory mechanism may help improve the reproductive potential of domestic animals.