INTRODUCTION
The growth of fish is affected by their specific genetics and various environmental factors, including water temperature, light, and nutrition. Light is the key factor that determines many behavior and ecological characteristics of fish and regulates various endocrine systems. The hormone melatonin, mainly secreted from the pineal organ, is highly sensitive to light and generally shows a daily secretion pattern with an increase in secretion during the night time and a decrease during the day time (Sanchez-Vazquez et al., 1997; Masuda et al., 2003; Rahman et al., 2004). Melatonin is synthesized via the activation of the arylalkylamine N–acetyltransferase (AANAT) enzyme. Melatonin secretion is dependent on the activation of AANAT and is involved in various physiological functions, including growth (Taylor et al., 2005; Vera et al., 2006; Falcon et al., 2007; De Pedro et al., 2008; Falcon et al., 2010).
The growth hormone (GH)-insulin-like growth factor (IGF) axis is well known to regulate growth in many teleost fish. GH secretion is induced by the stimulation of GH-releasing hormone, secreted in the hypothalamus. Meanwhile, another hypothalamic hormone, somatostatin (SS), acts mainly to inhibit the secretion of GH in the pituitary. Recent studies of teleost fish have established that SS is a main factor in inhibiting the secretion of GH (Rousseau et al., 2001; Very & Sheridan, 2002; Canosa et al., 2007). In addition, SS and GH are known to participate in various physiological functions, such as development, metabolism, immune response, and reproduction (Holstein & Cederberg, 1988; Poppinga et al., 2007; Zhang at al., 2009). SS genes exist in multiple isoforms in vertebrates. SS1 and SS2 are present in all vertebrates, and SS3, SS4 and SS5 are also found in some teleost fish. Furthermore, the SS6 gene has been reported in zebrafish (Liu et al., 2010). Each SS gene is synthesized from the precursor protein preprosomatostatin (PSS), of which various forms have been identified in some teleost fish (Liu et al., 2010).
The olive flounder (Paralichthys olivaceus) is a very commercially valuable fish species in the Korean aquaculture industry. Many studies are being conducted to improve the efficiency of olive flounder breeding and rearing (Cho et al., 2006; Park et al., 2012; Kim et al., 2015). The objective of the study is to explore the optimal photoperiod conditions for rearing olive flounder to understand their effect on the daily rhythms of growth-related genes (preprosomatostatin1, PSS1 and GH) and arylalkylamine N-acetyltransferase2 (AANAT2) mRNA. In addition, we examined how melatonin injections affected the expression of PSS1 and GH mRNA. Finally, we compared the change in body growth under different light conditions, including short-day and long-day conditions.
MATERIALS AND METHODS
Olive flounder were reared under a natural photoperiod and water temperature in an indoor concrete tank with a flow of seawater at the Marine Science Institute, Jeju National University. The fish were fed commercial pellets (Daehan Co., MP3, Busan, Korea) twice a day.
Fish (n=49, average body weight (BW) 45.2±0.7 g, average total length (TL) 17.1±0.1 cm) were acclimatized for 7 days in an indoor tank under a 12 h light:12 h dark photoperiod and natural water temperature conditions. Pelleted feed was provided twice a day at 08:00 h and 17:00 h for the first 6 days. After this time, fish were removed and tissue was sampled at 4 h intervals for 1 day at clock times 6, 10, 14, 18, 22, and 2 h. Before sampling, the fish were anesthetized using MS-222 and decapitated. The brain and pituitary tissues were collected and immediately stored at −80°C until analysis.
To determine the effect of melatonin on the expression of PSS1 and GH mRNA, fish were given an intraperitoneal injection (i.p.) of melatonin. Fish (n=12, average BW 153.1±3.4 g, average TL 24.7±0.2 cm) were separated into two groups and each group was reared for 3 days under 12 h:12 h conditions. After this time, the treatment group was injected with melatonin (Sigma, MO, USA) at 1 mg/kg ∙ BW using i.p. at 12:00. Sham group individuals were injected with saline (0.6% of NaCl) using i.p. at the same time. At 1 h after i.p., fish were anesthetized using the MS-222 and the brain and pituitary were extracted and stored at −80°C until analysis.
Fish (n=150, average BW 605.5±7.0 g, average TL 39.6±0.1 cm) were reared in an indoor tank with a flow of seawater under two different artificial photoperiod conditions, short day (SD, 10 h:14 h) and long day (LD, 14 h:10 h), for 16 weeks. Pelleted feed was supplied twice a day, at 08:00 h and 17:00 h, until the end of the experiment. During the experiment, the BW and TL of the fish were measured at 4 weeks intervals.
To perform PSS1 gene cloning in olive flounder, a degenerated primer set was designed on the basis of regions of high identity from PSS1 nucleotide sequences of other fish (GenBank accession numbers: Carassius auratus, CAU40754; Ctenopharyngodon idella, EU571475; Megalobrama pellegrini, AY247267). The olive flounder PSS1 cDNA was amplified by RT-PCR using a degenerate primer set (Table 1). PCR products were identified by electrophoresis on a 1% agarose gel followed by ethidium bromide staining and UV transillumination. The desired size band of PCR products was purified using a DNA purification kit (Promega, Madison WI, USA). Purified DNA fragments were cloned into the pGEM-T Easy Vector System (Promega) and transformed. Plasmid DNA was extracted according to the manufacturer's instructions for the Wizard® SV 96 Plasmid DNA Purification System (Promega, Madison, WI, USA). Extracted plasmid DNA was sequenced using a high-throughput DNA analysis system (Genotech, Korea). Fragment sequences of olive flounder PSS1 were confirmed by BLAST analysis. PSS1 full-length cDNA was obtained by RACE using the SMART RACE cDNA amplification kit (Clontech, Palo Alto, CA, USA) according to the manufacturer's instructions. The obtained cDNA was identified by electrophoresis of ethidium bromide on a 1% agarose gel, cloned with a T-blunt vector (Solgent, Daejeon, Korea), and sequenced (Genotech, Korea).
PSS1, preprosomatostatin1; GH, growth hormone; EF1-α, elongation factor 1-alpha.
Analysis of nucleotide sequences was performed using BLASTN (National Center for Biotechnology Information, National Institutes of Health, Bethesda, MD, USA). The signal peptides were identified with SignalP3.0 (www.cbs.dtu.dk/services/SignalP). Multiple alignment of amino acid sequences was analyzed using the ClustalW software (www.ebi.ac.uk/clustalw/). A phylogenetic tree was constructed by PHYLIP package (ver. 3.63, J. Felsenstein, University of Washington, Seattle, WA, USA).
For tissue-specific expression analysis of PSS1 mRNA, brain tissue was divided into five portions: telencephalon, optic tectum, diencephalon, cerebellum, and medulla oblongata. Further pituitary and other peripheral tissues (heart, kidney, liver, intestine, spleen, gonad, and muscle) were also analyzed. PSS1 mRNA expression was confirmed using the RT-PCR and real-time quantitative PCR (real-time qPCR) analysis.
For extraction of total RNA, brain and pituitary tissues were absolutely homogenized with RNAiso Plus (Takara Bio Inc, Otsu, Japan) reagent. Total RNA was extracted following the manufacturer's protocol and total RNA concentration were measured using the Nano Vue (GE Healthcare, ver. 1.0.1, UK). Total RNA was treated using the RQ1 RNase-Free DNase (Promega, Madison, WI, USA) and cDNA was synthesized using 500 ng of total RNA and A260/280 ratio of 1.8–2.0 with the PrimeScript RT reagent kit (Takara Bio Inc. Otsu, Japan). The synthesized cDNA was used in the analysis of PSS1 gene cloning and real-time qPCR.
For RT-PCR and real-time qPCR, PSS1 primers were designed using the isolated PSS1 gene nucleotide sequence in olive flounder. GH primers were designed using information from the NCBI web-site (Table 1). The RT-PCR conditions consisted of a denaturation cycle of 94°C for 2 min, followed by 35 PCR cycles each consisting of 45 s denaturation at 94°C, 45 s annealing at 55°C, and 1 min extension at 72°C. Extension time in the last cycle was elongated to 3 min. The obtained PCR products were electrophoresed with ethidium bromide on 2% agarose gel and visualized on a UV transilluminator. Negative controls were examined for each primer whenever cDNA was excluded from the RT-PCR.
Real-time qPCR was performed using the CFXTM Real-time System (Bio-Rad, Hercules, CA, USA) with Evagreen premix PCR kit (ABM Inc. Canada). Real-time qPCR amplification was performed by initial denaturation at 95°C for 10 min, 40 cycles of 95°C for 15 s, 60°C for 1 min, and 60°C for 1min. The EF1-α (elongation factor 1-alpha) gene was used as a reference gene.
All gene expression data from real-time qPCR were expressed as mean±SE (SEM). All statistical differences among means were analyzed by one-way analysis of variance (ANOVA) followed by Duncan's multiple range test and independent sample t-tests using Statistics 21.0 for Windows (SPSS Inc.).
Results
We isolated the PSS1 full sequence information from the brain tissue and registered a PSS1 sequence with NCBI (accession number AB693833). The PSS1 sequence totaled 638 bp fragments and consisted of a 96-bp 5’-untranslated region (UTR), a 363-bp open reading frame (ORF), and a 179-bp 3’-UTR (Fig. 1). In addition, a signal peptide consisting of 26 amino acids was identified and 14 SS-14-1 amino acids were identified in C-terminal (Fig. 1). In addition, the results of multiple alignment between olive flounder PSS1 cDNA and PSS1 cDNA in other fish all matched the 14 amino acids of SS-14-1 (Fig. 2).
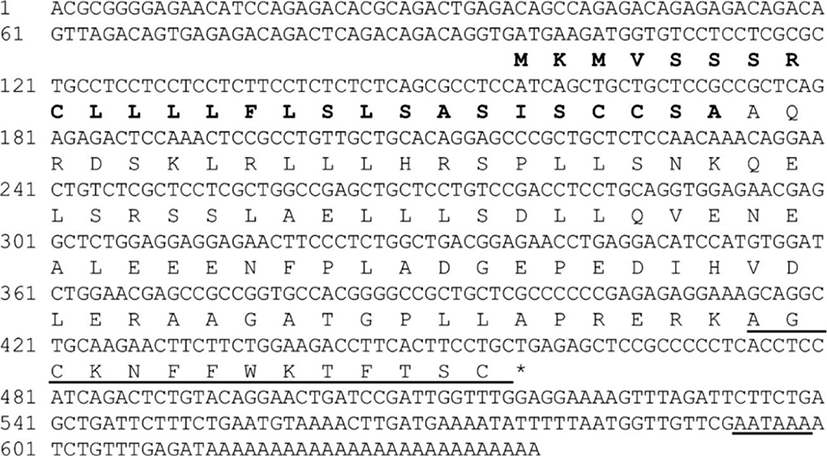
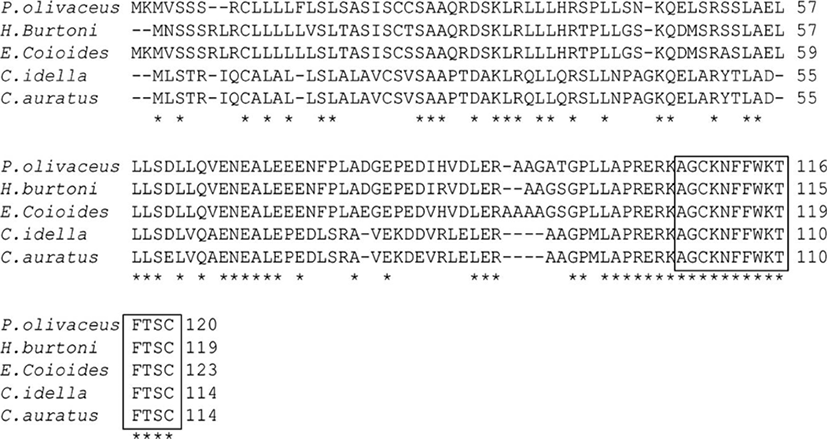
The identity analysis results between olive flounder PSS1 and PSS1 from other fish showed 45% to 86% agreement (Table 2).
PSS1, preprosomatostatin1.
Phylogenetic analysis revealed that the olive flounder PSSⅠ was grouped with Haplochromis burtoni (Fig. 3).
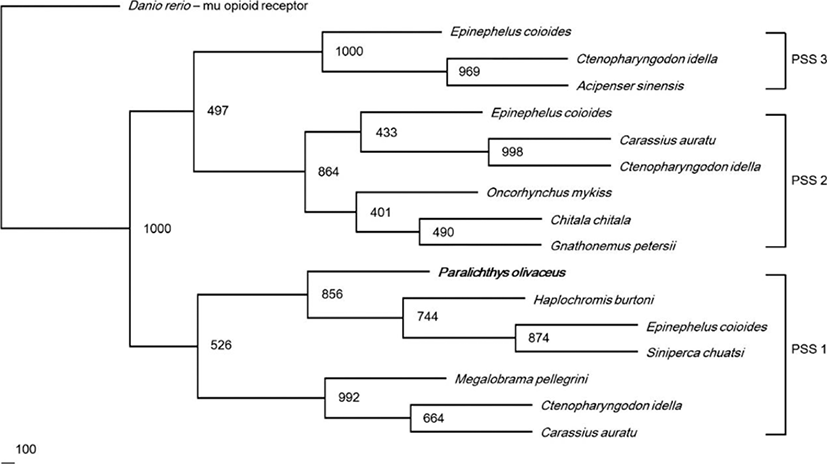
We observed the tissue distribution and relative expression of PSS1 mRNA using RT-PCR and real-time qPCR (Fig. 4). The RT-PCR analysis showed that, the expression band of PSS1 mRNA was detected in the telencephalon, optic tectum, diencephalon, and medulla oblongata of the brain. Among the peripheral tissues, weak expression of PSS1 mRNA was detected in the gonads (Fig. 4a). Real-time qPCR analysis revealed that the highest level of expression of PSS1 mRNA was detected in the telencephalon. PSS1 mRNA expression was also detected in the diencephalon, optic tectum, and medulla oblongata (Fig. 4b).
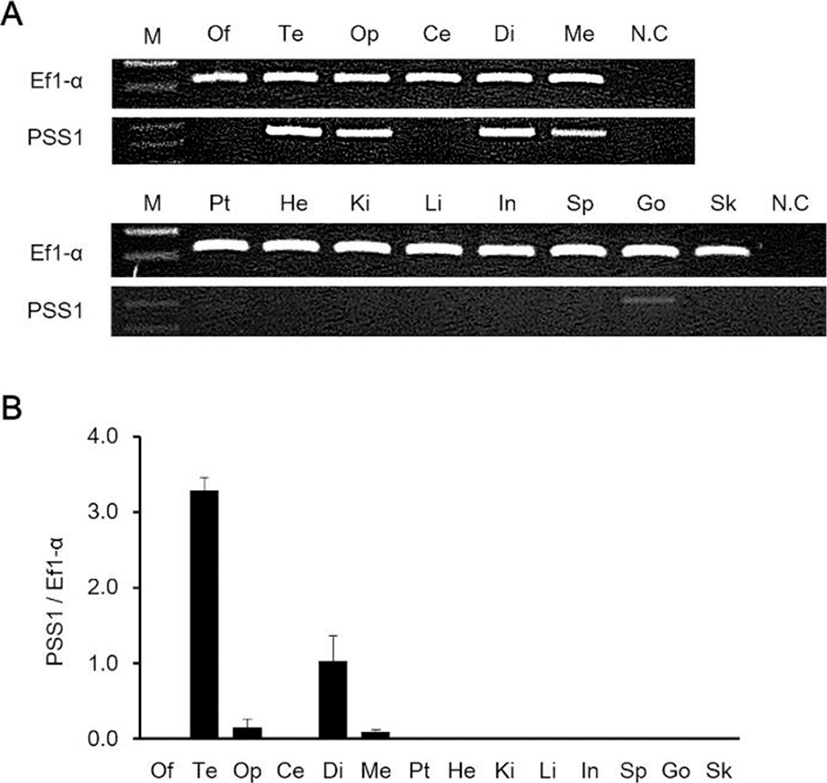
We investigated the daily variations of AANAT2, PSS1 and GH mRNA expression in the brain and pituitary under 12 h:12 h conditions, and the mRNA expression of all genes was higher at night-time than during the day (Fig. 5). The expression of AANAT2 and PSS1 mRNA in the brain continued at low levels from CT6 to CT14, but, the expression was highest at CT22 (Figs. 5a and 5b). The expression of GH mRNA in the pituitary remained steady at low levels from CT6 to CT22 and rapidly increased at CT2 (Fig. 5c).
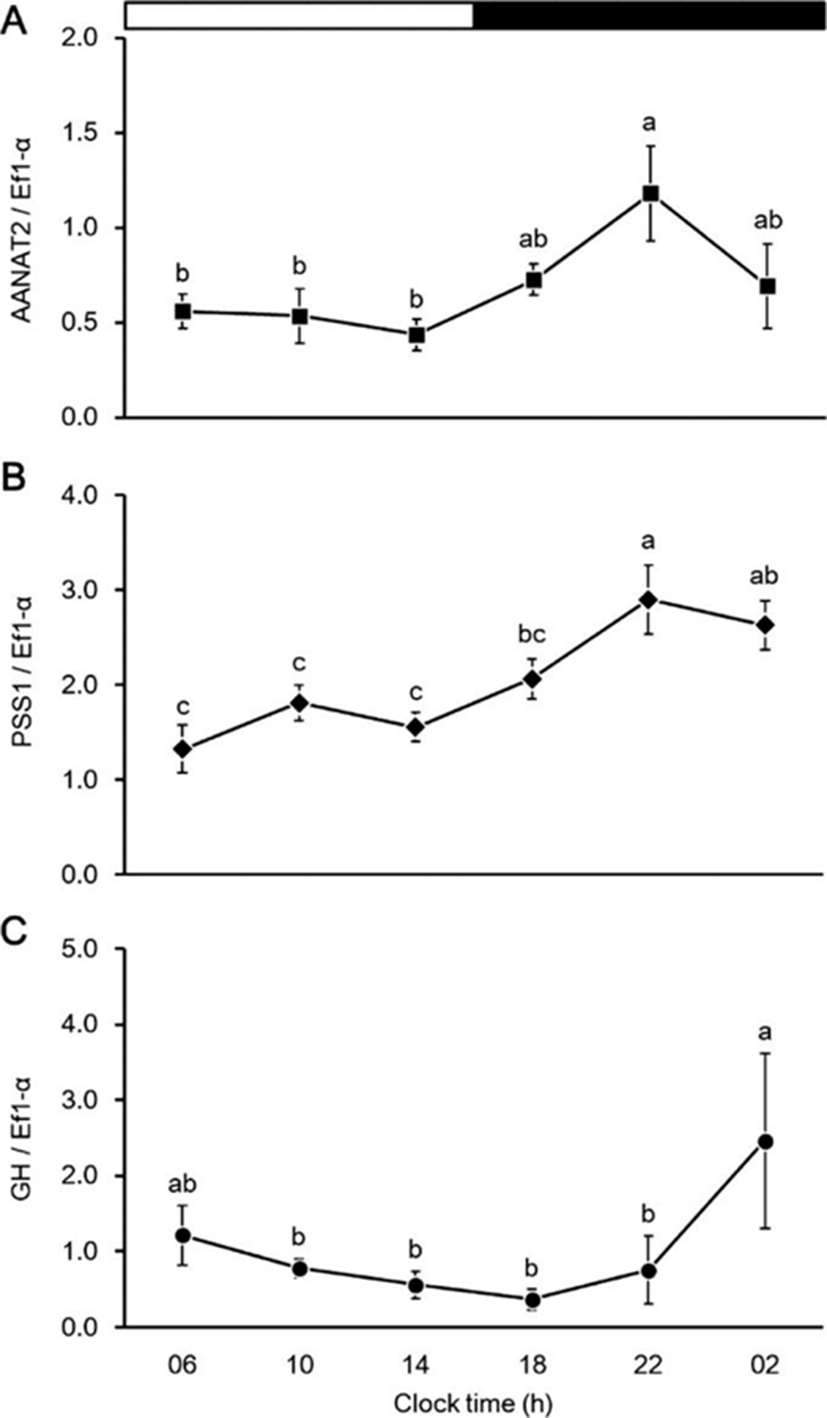
The effects of melatonin injection on the expression of PSS1 and GH mRNA in the brain and pituitary, respectively, shown in Fig. 6. The PSS1 mRNA expression in the brain did not differ between the treatment and sham groups (Fig. 6a). However, the GH mRNA expression in the pituitary was significantly higher in the treatment group than in the sham group (Fig. 6b).
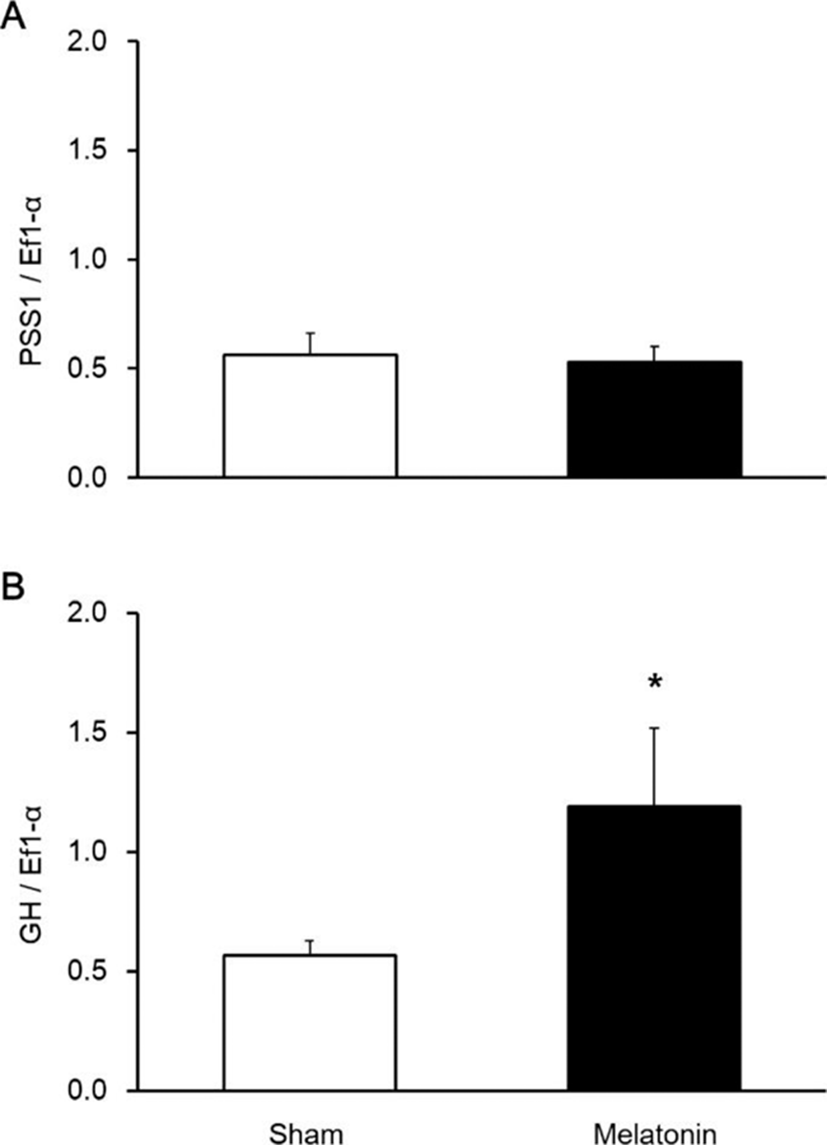
The fish were reared for 16 weeks under SD and LD conditions, and the changes in body growth during this period are shown in Fig. 7. The BW showed no significant difference between the two groups until 12 weeks; by 16 weeks, the BW of the SD group was significantly higher than in the LD group (p<0.05; Fig. 7a).
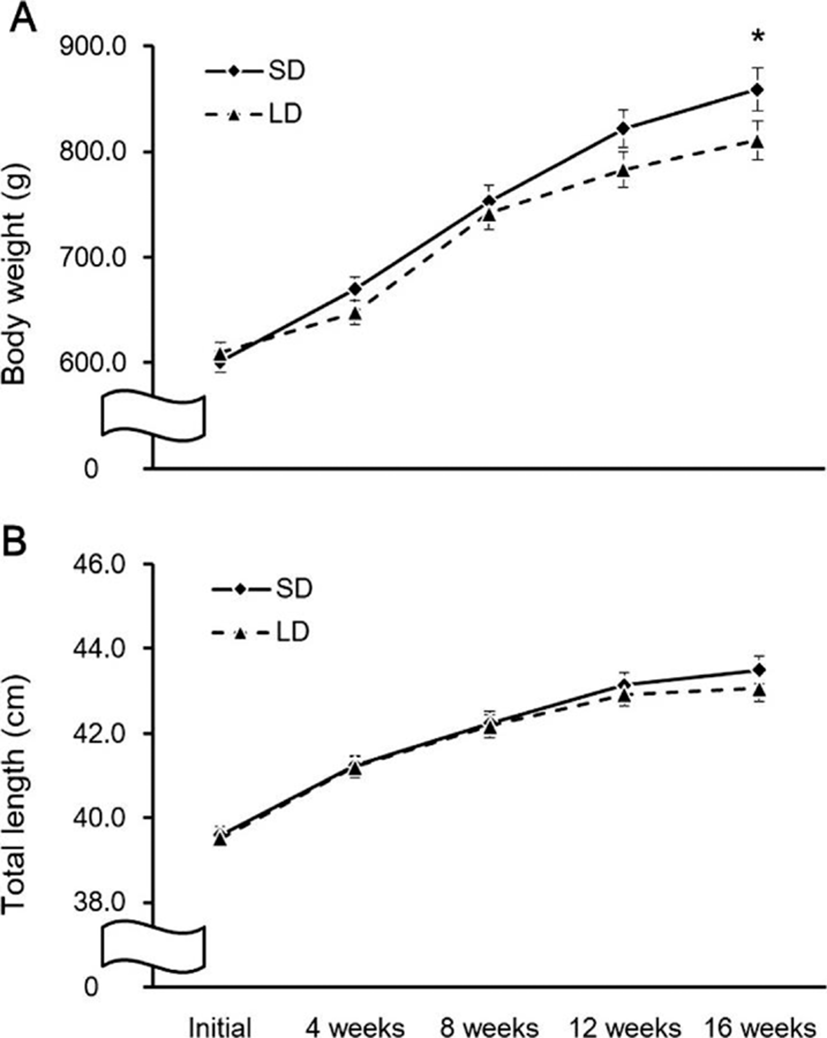
Discussion
We investigated the daily variations in AANAT2, PSS1, and GH mRNA expression in the brains and pituitary glands of olive flounder. Variations in melatonin secretion or AANAT mRNA expression have been reported in many teleost fish (Migaud et al., 2006; Nikaido et al., 2009; Falcon et al., 2010; Seth & Maitra, 2010; Liu et al., 2019). In a recent study of air-breathing catfish (Clarias gariepinus), AANAT2 mRNA expression followed a circadian rhythm (Singh et al., 2017). In the present study, increased AANAT2 mRNA expression occurred during the dark phase relative to the light phase, and this expression pattern was also found under a continuous dark condition. Although many studies have examined melatonin variation, few have focused on the study of the variation pattern of SS, and only a few have reported that SS levels in plasma are higher at night than during the day in the rats (Peinado et al., 1990; Ishikawa et al., 1997). In one of our previous studies, we studied the variation patterns of melatonin secretion and SS mRNA expression in tiger puffer (Takifugu rubripes), observed that the melatonin secretion and SS1 mRNA expression increased at night relative to its level during the day under a 12 h:12 h photoperiod (Kim et al., 2017). The variation pattern of GH secretion is known through studies of some teleost fish (Holloway et al., 1994; Gomez et al., 1996). For instance, the daily expression patterns of GH mRNA were observed in rabbitfish (Siganus guttatus) (Ayson & Takemura, 2006). According to that study, the GH mRNA expression was significantly higher during the dark phase than during the light phase under 12 h:12 h photoperiod. However, this expression pattern disappeared under continuous light and continuous darkness, and these results suggested that the expression of GH mRNA is regulated according to the light and dark cycle. In the present study, the variations in the expression of AANAT2, PSS1, and GH mRNA in olive flounder was similar to that observed in studies on other fish. Therefore, expression of AANAT2, PSS1, and GH mRNA in olive flounder follow a nocturnal rhythm. Our study did not determine whether this nocturnal rhythm was caused by the presence/absence of light or by a circadian rhythm unrelated to light, so we recommend further study of the circadian rhythm.
We investigated the effect of melatonin on the expression of PSS1 and GH mRNA to explore the correlation between melatonin and PSS1 and GH genes. Few previous studies have examined the relationship between melatonin and PSS1 and the only species used in such studies were rat and tiger puffer. Examination of the hippocampus of rats showed that melatonin treatment caused a decline in somatostatin-like immunoreactivity, as well as in the number of SS receptors, but chronic treatment with melatonin did not result in SS inhibition (Izquierdo-Claros et al., 2004). In tiger puffer, melatonin treatment did not change the expression of SS mRNA in the brain (Kim et al., 2017). In the present study, melatonin treatment by i.p. did not results in activation of PSS1 mRNA expression in the brain, a result that was similar to that found in tiger puffer. Thus, we suggest that melatonin probably does not directly affect the expression of SS mRNA in the brain of olive flounder.
However, the expression of GH mRNA in the pituitary did increase after melatonin treatment. Melatonin is a major factor with various physiological effects in fish, but few studies have examined the effects of melatonin on GH secretion in the pituitary glands of fish. An in vivo experiment with rainbow trout (Oncorhynchus mykiss) observed that GH secretion in the pituitary increased after melatonin treatment in cultured trout, but also observed that GH secretion was inhibited under specific pharmacological conditions (Falcon et al., 2003). The author concluded that melatonin may have a bimodal effect that either induces or inhibits GH secretion in the pituitary. Our experimental result suggested that, melatonin affects the activation of GH mRNA in olive flounder. Nocturnal variation in GH mRNA expression in the pituitary appears to be synchronized and is affected by the nocturnal variation of AANAT2 mRNA expression in the brain, whereas the nocturnal variation of PSS1 mRNA expression in the brain appears to have its own variation pattern, independent of AANAT2 mRNA expression.
Photoperiod is a very important environmental factor affecting the physiology of fish, especially their growth and reproduction. Most studies of the effect of photoperiod on growth in various fish species reported that a LD condition enhanced the growth of fish (El-Sayed & Kawanna, 2004; Gines et al., 2004; Carrillo et al., 2010; Kitagawa et al., 2015). For example, red sea bream (Pagrus major) that were reared under various photoperiod conditions (6 h:6 h, 12 h:12 h, 16 h:8 h, and 24 h:0 h) showed enhanced growth performance under 16 h:8 h and 24 h:0 h photoperiods and improved food intake, feed efficiency, and digestibility compared to other photoperiod conditions (Biswas et al., 2005). Blunt snout bream (Megalobrama amblycephala) that were reared under different photoperiod conditions (8 h:16 h, 12 h:12 h, and 16 h:8 h) showed significantly enhanced growth performance under a long photoperiod, as well as increased expression of GH and IGF-1 mRNA in the brain and liver, respectively (Tian et al., 2019).
However, our findings conflict with these results. In the present study, the olive flounder gained more weight under the SD condition than the LD condition. Interspecies differences in fish may be due to different feeding states, growth stages and, photoperiod conditions in various experiments. In European sea bass (Dicentrarchus labrax), plasma melatonin levels were affected by different photoperiods, and the duration of high secretion of melatonin at night was increased when night time was extended, suggesting that the melatonin secretion is regulated by photoperiod (Bayarri et al., 2004). Thus, in the present study, the SD condition that induced weight gain in olive flounder was probably a result of prolonged high melatonin secretion and GH mRNA expression due to extended night time. However, this hypothesis is difficult to prove without additional data, and further study is needed of the characteristics of melatonin secretion and GH mRNA expression due to photoperiod change.
In conclusion, we investigated the hypothesis that melatonin produced at night regulates the expression of growth-related genes (PSS1 and GH) and affects the body growth of olive flounder. As a result, the expression of AANAT2, PSS1, and GH mRNA showed nocturnal variations, and melatonin injection was able to induce higher expression of GH mRNA in the pituitary. Olive flounder gained more weight under the SD condition than under the LD condition. Therefore, we recommend that aquaculture facilities rearing olive flounder use a photoperiod with a long night-time to improve body growth, but the mechanism underpinning the effects of melatonin on growth-related genes remains unclear. The present study provides basic data on environmental conditions for efficient aquaculture practices.