INTRODUCTION
The family of glycoprotein hormone receptors including lutropin chorionic gonadotropin hormone receptor (LH/CGR) belongs to the superfamily of G protein-coupled receptors (GPCRs) that couple extracellular agonists to intracellular effector molecules through the reactions of heterotrimeric G proteins and arrestins (Pierce et al., 2002; Martemyanov & Garcia-Maarcos, 2018; Wan et al., 2018; Seong et al., 2020; Jones et al., 2021). A β-arrestin recruitment to ligand-stimulated GPCRs promotes non-canonical signaling cascades follow by GPCR desensitization and endocytosis (Beautrait et al., 2017; Pakharukova et al., 2020; Slosky et al., 2020).
GPCR internalization is a specific process triggered by agonist stimulation, following desensitization of the signaling function of receptors, and increases cAMP responsiveness (Min et al., 1998; Kim et al., 2019; Byambaragchaa et al., 2020; Min et al., 2021). Protein phosphorylation sites in various signaling pathways are important in regulating cell proliferation (Seong et al., 2020). The gonadotropin hormone receptors, LH/CGR, and follicle-stimulating hormone receptor (FSHR) in humans, rats, mice, and horses are highly conserved regions within the transmembrane helices (Min et al., 1998; Zhang et al., 2007; Byambaragchaa et al., 2018).
In the carboxyl (C)-terminal deleted mutant, the rat LHR (rLHR)-5S/T→A mutant was considerably decreased in cAMP responsiveness, and the rate of internalization was significantly slower compared to the wild-type rLHR (rLHR-wt) (Wang et al., 1997). Two deleted mutants (rLHR-t628 and rLHR-t631) in the rLHR C-terminus are involved in the protein kinase A (PKA) signaling pathway, desensitization, and downregulation by phorbol 12-myristate-13-acetate (PMA) and human chorionic gonadotropin (hCG) treatment (Sánchez-Yagüe et al., 1992; Wang et al., 1996). Recently, we also reported that EC50 and Rmax levels of the equine FSHR (eFSHR)-t641 mutant, which removed 10 potential phosphorylation sites, were 0.58-fold and 68.4% of that in eFSHR-wt (Seong et al., 2020). The EC50 level of eel FSHR-t614 mutant, in which 10 phosphorylation sites in the C-terminal region were deleted, was approximately 60.4% in Chinese hamster ovary (CHO) -K1 cells, and the maximal response was drastically lower than that of eel FSHR-wt (Kim et al., 2018). However, this mutant showed a similar cAMP response in PathHunter Parental cells expressing β-arrestin, despite being slightly lower. Thus, the deletion of phosphorylation sites in the C-terminal intracellular region is extremely important for preventing phosphorylation post-translation and retarding or preventing hCG- or PMA-induced uncoupling.
Other studies have shown that agonist-induced activation and phosphorylation of rFSHR are not essential for internalization (Nakamura et al., 1998). However, the rFSHR-t635 mutant contains only one potential phosphorylation site in the intracellular region and responds to increases in the cAMP response (Hipkin et al., 1995a), demonstrating that the mutant is not inhibited in the signal transduction pathway of phosphorylation or uncoupling (Ascoli, 1996). With respect to the truncation of phosphorylation sites, a dramatical loss of cAMP responsiveness in hFSHR-t678 has been reported (Krishnamurthy et al., 2003; Bhaskaran & Ascoli, 2005), the hLHR-t682 mutant is slower at the cell surface loss of the receptor than that of hLHR-wt (Bhaskaran & Ascoli, 2005), and the hLHR-t682 complex with hCG is routed to a lysosomal degradation pathway (Kishi et al., 2001; Hirakawa and Ascoli, 2003; Galet et al., 2004).
Therefore, the C-terminal region of eLH/CGR has 14 potential phosphorylation sites and a highly conserved leucine motif that is essential for trafficking (Duvernay et al., 2004; Byambaragchaa et al., 2021a,b,e). The eCG, secreted from the placenta during early pregnancy, is a unique molecule that displays both LH- and FSH-like activities in non-equid species (Min et al., 2019, 2020, 2021; Lee et al., 2021) and exhibits only LH-like activity in equine species (Lee et al., 2017, 2021; Park et al., 2017; Min et al., 2019, 2020; Byambaragchaa et al., 2021c,d).
Thus, the C-terminal region containing the phosphorylation sites in eLH/CGR must be determined for the elucidating the signal transduction and downregulated pathways. To characterize the signal transduction of the β-arrestin function through the eLH/CGR-eCG complex, we analyzed the agonist-stimulated cAMP response of both eLH/CGR-wt and eLH/CGR-t656.
MATERIALS AND METHODS
The oligonucleotides used in this study were synthesized by Genotech (Daejeon, Korea). The cloning vector (pGEMT-T easy) was purchased from Promega (Madison, WI, USA). The pcDNA3 expression vector, pCMV-ARMS1-PK2 expression vector, Freestyle MAX reagent, FreeStyle CHO-suspension (CHO-S) cells and AssayComplete medium were purchased from Invitrogen (Carlsbad, CA, USA). The PathHunter Parental CHO-K1 cell line expressing β-arrestin 2 was purchased from DiscoveRx (San Diego, CA, USA). The pCORON1000 SP VSV-G-tag expression vector was purchased from Amersham Biosciences (Piscataway, NJ, USA). The PMSG-ELISA kit was purchased from DRG International (Mountainside, NJ, USA). Restriction enzymes and DNA ligation reagents were purchased from Takara Bio (Shiga, Japan). Fetal bovine serum (FBS) was obtained from HyClone Laboratories (Logan, UT, USA). The cAMP Dynamic 2 immunoassay kit was purchased from Cisbio (Codolet, France). CHO-K1 cells and HEK 293 cells were obtained from the Korean Cell Line Bank (KCLB, Seoul, Korea). The QIAprep-Spin plasmid kit was purchased from Qiagen (Hilden, Germany). All other reagents were obtained from Sigma-Aldrich (St. Louis, MO, USA).
The eLH/CGR cDNA was cloned using cDNA from equine testes and ovaries, as previously reported (Seong et al., 2020; Byambaragchaa et al., 2021). Polymerase chain reaction (PCR) fragments of the C-terminal deleted mutant were cloned into pGEMT and sequenced. The full fragments of the eLH/CGR-wt and eLH/CGR-t656 mutants were ligated into the pcDNA3 mammalian expression vector cut by the EcoRI and XhoI sites. For the PathHunter Parental CHO-K1 cells, eLH/CG cDNA digested using Nhe1 and Sac1 was cloned into the same enzyme sites as the pCMV-ARMS1-PK2 expression vector, as previously described (Seong et al., 2020). The eLH/CGR-wt and eLH/CGR-t656 mutants were subcloned into the pVSVG expression vector using XhoI and EcoRI and the entire region of the deleted mutant was confirmed by DNA sequencing. Fig. 1 presents a schematic representation of the eLH/CGR intracellular region and the truncated mutant, in which the potential phosphorylation sites were deleted after amino acid residue 656. There was no stop codon in the C-terminal region (Sac1 enzyme site) of the pCMV-ARMS1-PK2 expression vector. The direction was confirmed by restriction mapping and verified by sequencing the entire open reading frame. Fig. 2 shows the PCR products of eLH/CGR full-length and t656 mutant cDNAs, and these receptor fragments were cloned into each expression vector, pcDNA3, pVSVG, and pCMV-ARMS1-PK2.
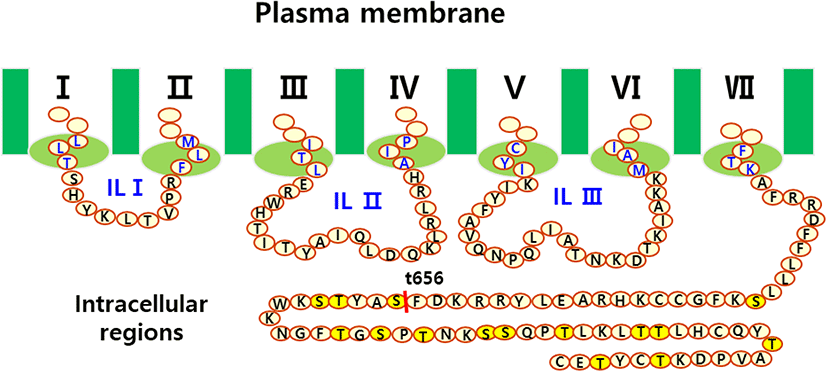
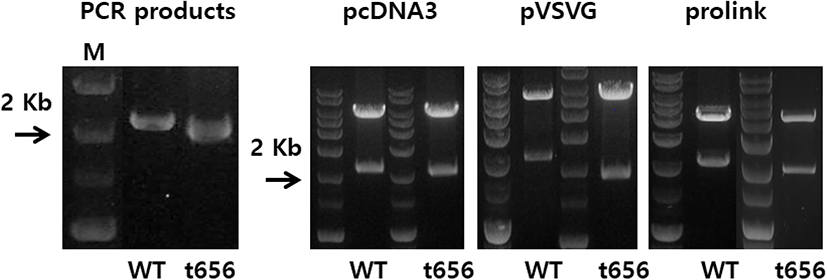
For rec-eCG production, the vectors were transfected into CHO-S cells using FreeStyleTM MAX reagent, as previously described (Byambargachaa et al., 2021e). The CHO-S cells were cultured in FreeStyle CHO expression medium at 1×107 cells per 30 mL of medium for 3 days. After transfection with the expression vectors, the culture medium was collected on Day 7 post-transfection and centrifuged at 100,000×g for 10 min at 4℃. The supernatant was collected and concentrated using a Centricon filter. Finally, the sample was mixed 10–20 times and the quantity of rec-eCG was determined using ELISA as previously reported (Byambaragchaa et al., 2021c,d).
CHO-K1 cells were cultured in a growth medium [Ham’s F-12 medium containing antibiotics (penicillin and streptomycin), glutamine (2 mM), and 10% FBS]. PathHunter Parental cells were engineered to stably express an enzyme acceptor-tagged β-arrestin fusion protein. These cells were transiently transfected according to the supplier’s protocol as previously described (Seong et al., 2020). The CHO-K1 cells and PathHunter Parental cells were grown to 80%–90% confluence in 6-well plates. The diluted plasmid DNAs were transfected using Lipofectamine reagent. After 5 min, growth medium containing 20% FBS was added to each well. Transfected cells were adjusted for cAMP analysis 48–72 h post-transfection.
cAMP accumulation in the CHO-K1 cells and PathHunter Parental cells expressing eLH/ CGR-wt and eLH/CGR-t656 mutants was measured using cAMP Dynamics 2 competitive immunoassay kits as described previously (Byambaragchaa et al., 2021e). The cAMP response assay used a cryptate-conjugated anti-cAMP monoclonal antibody and a d2-labeled cAMP reagent. Transfected cells were seeded in a 384-well plate at 10,000 cells per well. Compound medium buffer containing the ligand (5 μL) was added to each well and incubated for 30 min. Subsequently, cAMP-d2 and anti-cAMP-cryptate were added to each well and incubated for 1 h at room temperature. The plate was detected by measuring compatible homogeneous time-resolved fluorescence (HTRF) energy transfer (665 nm/620 nm) using a TriStar2 S LB942 mi microplate reader (BERTHOLD Tech., Wildbad, Germany). The results were represented as Delta F% (cAMP inhibition), which was calculated as [Delta F% = (Standard or sample ratio-Sample negative) × 100/ Ratio negative]. The cAMP concentration for Delta F% values was calculated using the GraphPad Prism software (version 6.0; GraphPad Software, La Jolla, CA, USA).
RESULTS
As a type of GPCR, eLH/CGR-wt has a long C-terminal region containing approximately 14 phosphorylation sites. As shown in Fig. 1, potential phosphorylation sites were deleted by PCR to assess the function of phosphorylation in signal transduction through cAMP responsiveness. The eLH/CGR-t565 mutant was deleted from the 45 amino acids containing 14 phosphorylation sites in the C-terminal region of eLH/CGR. The PCR results for the eLH/CGR-wt and eLH/ CGR-t656 mutants are shown in Fig. 2. The full fragments of the eLH/CGR-wt and eLH/ CGR-t656 mutants were ligated into the pcDNA3 and pVSVG mammalian expression vectors to be expressed in CHO-K1 cells. The eLH/CG cDNAs were cloned into the pCMV-ARMS1-PK2 expression vector to be expressed PathHunter Parental CHO-K1 cells. Finally, we constructed six expression vectors: pcDNA3-eLH/CGR-wt, pcDNA3-eLH/CGR-t656, pVSVG-eLH/CGR-wt, pVSVG-eLH/CGR-t656, pCMV-ARMS1-PK2-eLH/CGR-wt, and pCMV-ARMS1-PK2-eLH/CGR-t656.
The eLH/CGR-wt and eLH/CG-t656 plasmids were transfected into CHO-K1 cells and PathHunter Parental cells expressing β-arrestin. The activity of cAMP responsiveness is expressed as Delta F%, as shown in Fig. 3. The standard curve was appropriately inhibited by increasing the concentration (0.17–712 nM). Receptor cells were treated with different concentrations (0.008–1,500 ng/mL) of rec-eCG. The Delta F% values were estimated to express the cAMP concentration (nM). The cAMP values increased in a dose-dependent manner relative to the ligand concentration in both CHO-K1 cells and PathHunter Parental cells (Fig. 4).
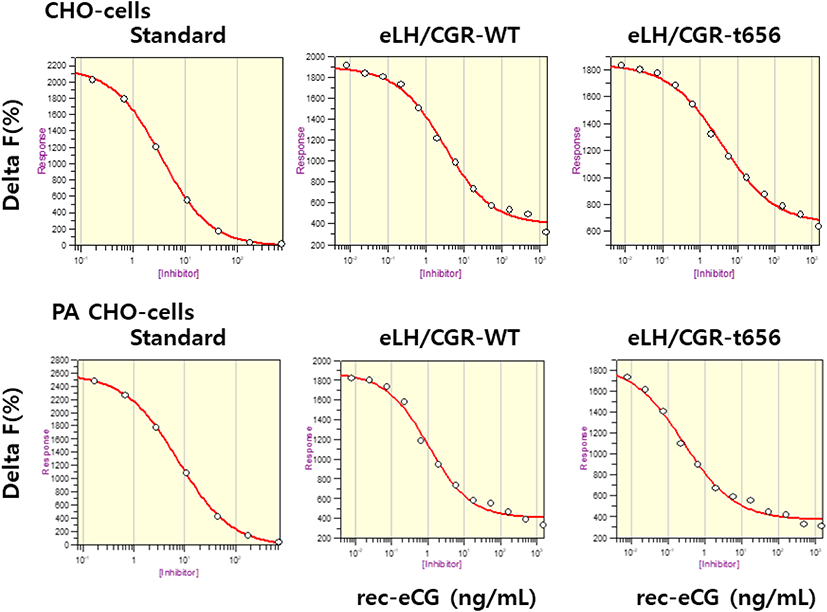
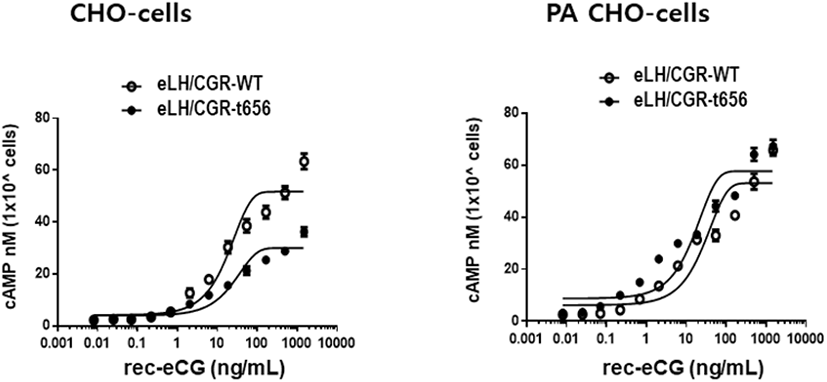
In the CHO-K1 cells, the EC50 values of eLH/CGR-wt and eLH/CGR-t656 mutant was 18.7 ng/mL and 25.9 ng/mL, respectively. The activities of both receptors were approximately 72.2% in the eLH/CGR-t656 mutant compared to that in the eLH/CGR-wt. The Rmax level in the eLH/CGR-t656 mutant was 0.57-fold lower than that of the eLH/CGR-wt as shown in Table 1. Therefore, the C-terminal phosphorylation regions were critical for the signal transduction of cAMP responsiveness in CHO-K1 cells expressing the deleted receptor mutant. However, the EC50 value in the PathHunter Parental cells transfected with eLH/CGR-wt and eLH/CGR-t641 was 27.6 ng/mL and 15.9 ng/mL, respectively (Table 1). Truncation of the C-terminal region was approximately 1.78-fold higher than that of the wild type receptor. However, eLH/CGR-wt and eLH/CGR-t656 mutants had similar Rmax levels. Thus, the EC50 level and maximal response in CHO-K1 cells expressing the eFSHR-t656 mutant were remarkably lower than those in cells expressing eLH/CGR-wt. However, the results in PathHunter Parental cells displayed different patterns, indicating that the eLH/CGR-t656 mutant was considerably high. This suggests that phosphorylation sites in the eLH/CGR C-terminal region play a pivotal role in signal transduction in CHO-K1 cells. The decreased cAMP and Rmax levels in the C-terminal-deleted mutant were recovered as eLH/CGR-wt in PathHunter Parental cells. We believe that the expression of β-arrestin in PathHunter Parental cells is a major factor for increasing the EC50 value and Rmax level in the eLH/CGR-t656 mutant.
Values are the means±SEM of triplicate experiments. Log (EC50) values were determined from the concentration-response curves from in vitro bioassays.
DISCUSSION
GPCR signals through coupling of G proteins and of β-arrestins alternatively activate beta-adrenergic-receptor kinase (βARK) and G-protein-coupled kinase (GRK). The second messenger kinases of βARK and GRK phosphorylate serine and/or threonine residues in the intracellular region of GPCRs following agonist-induced treatment (Kim et al., 2018). We and others have shown that ligands (hCG, eCG, eFSH, eel FSH, and eel LH) of glycoprotein hormone receptors result in the accumulation of cAMP responses (Hipkin et al., 1995a,b; Min et al., 2004; Bhaskaran & Ascoli, 2005; Kim et al., 2018; Byambaragchaa et al., 2021c,d, 2022). Several features of the post-endocytotic trafficking of receptors (mouse, rat, and human) that demonstrate routing of most internalized receptors to the lysosomes, and degradation of hormone-receptor complexes have been elucidated (Baratti-Elbaz et al., 1999; Kishi et al., 2001; Bhaskaran & Ascoli, 2005).
We analyzed the roles of phosphorylation sites in the uncoupling of eLH/CGR from cAMP accumulation in cells expressing eLH/CGR-wt and eLHCGR-t656. We tested the function of β-arrestin in the phosphorylation sites of the PathHunter Parental CHO-K1 cells expressing β-arrestins. In this present study, the C-terminal phosphorylation sites were critical for signal transduction of cAMP responsiveness in CHO-K1 cells. The EC50 value in the truncated potential phosphorylation sites of the C-terminal cytoplasmic tail was approximately 72% of that in eLH/ CGR-wt. The maximal response also dramatically decreased, similar to the EC50 value of the C-terminal deletion in eLH/CGR. These results are consistent with our previous data, indicating that the truncated phosphorylated sites in the cAMP response of eel FSHR-t614 decreased considerably to 60.4% of that of eel FSHR-wt, demonstrating that the maximal response of eel FSHR also decreased significantly by C-terminal deletion (Kim et al., 2018).
Other groups have reported significant phosphorylation sites in the signal transduction of receptors. The change of serine and threonine to alanine in the potential phosphorylation sites of rLH/CGR considerably reduces cAMP responsiveness (Wang et al., 1997). The truncated mutants (rLH/CGR-t631 and rLH/CGR-628) showed a delay in the early phase of desensitization, complete loss of PMA-induced desensitization, and an increased rate of downregulation (Wang et al., 1996). A deletion mutant at rLH/CGR-t631 impaired receptor phosphorylation. However, the cAMP response to Cholera toxin was also slightly elevated, and the Rmax level was 1.5-fold higher than that of wild-type receptor (Hipkin et al., 1995b). These findings suggest that the deleted mutant at the 631 amino acid of rLH/CGR is not phosphorylated and does not arise from insufficient hormone binding. Thus, our results in CHO-K1 cells suggest its primary role in post-translational phosphorylation.
In eFSHR, we also reported that the EC50 value and Rmax level in the eFSHR-t641 mutant were 0.58-fold lower than and 66% of that observed in eFSHR-wt in PathHunter Parental CHO-K1 cells (Seong et al., 2020). In this study, eLH/CGR deletion mutant recovered as much as the wild-type receptor. This may not have caused the deletion of the phosphorylation sites in the C-terminal region. One amino acid (serine) of the potential phosphorylation sites remained in eLH/CGR-t656, as shown in Fig. 1. The eFSHR-t641 deleted all potential phosphorylation sites in the intracellular region (Seong et al., 2020). Thus, we suggest that the phosphorylation sites in the C-terminal region of glycoprotein hormone receptors are indispensable for signal transduction through their receptor-ligand complex.
Additionally, β-arrestin, one of the main signal transduction pathways, is considered an important factor, indicating that cAMP and EC50 levels in the eLH/CGR-t656 mutant were restored to levels similar to those of the wild-type receptor in PathHunter Parental CHO-K1 cells. Our observations in cells expressing the β-arrestin kinase are not consistent with that in prior studies, indicating that phosphorylation sites are essential for signal transduction through G proteins. We hypothesize that a single phosphorylation site in the C-terminal region of eLH/CGR affects the post-translational phosphorylation of LH/CGRs, even though we have not analyzed the lack of phosphorylation in eLH/CGR-t656. The hFSHR-t678 mutant showed a greater degree of downregulation of cell surface receptors than that of hFSHR-wt in human embryonic kidney 293 (HEK-293) cells (Krishnamurthy et al., 2003). The hFSHR-t678 in mouse granulosa cells displayed approximately 60% loss of cell surface receptors despite approximately 40% loss of cell surface receptors in hFSHR-wt (Bhaskaran & Ascoli, 2005; Galet et al., 2003). These results are inconsistent with our results in PathHunter Parental CHO-K1 cells expressing β-arrestin 2 kinase. Thus, we suggest that eLH/CGR at the phosphorylation sites of the C-terminal cytoplasmic tail is necessary for signal transduction.
Recently, we also reported that eel FSHR-t635, in which all potential phosphorylation sites in the intracellular cytoplasmic tail were deleted, displayed an EC50 of approximately 60.4% of that of eel FSHR-wt (Kim et al., 2018). Although the rFSHR-truncated mutant did not affect phosphorylation or uncoupling (Hipkin et al., 1995a), the eFSHR-t641 in our study was considerably affected by cAMP responsiveness in cells expressing eFSHR-t641 with β-arrestin (Seong et al., 2020). Our data in the present study are consistent with that in our previous study, indicating that the deletion mutant of the C-terminal region greatly decreased the cAMP response.
In conclusion, eLH/CGR-wt in CHO-K1 cells responded to increased cAMP levels in a dose-dependent manner. The cAMP-mediated receptor was greatly reduced in the eLH/CGR-t656mutant that lacked phosphorylation sites, but one site of potential phosphorylation remained. The cAMP responsiveness in PathHunter Parental CHO-K1 cells was restored to the same level as the wild-type receptor. Thus, we suggest that C-terminal phosphorylation sites in eLH/CGR are necessary for signal transduction. Further studies are required to elucidate the signaling mechanisms that regulate internalization, loss of cell surface receptor, mitogen-activated protein (MAP) kinase, extracellular regulated kinase (ERK), and recycling in PathHunter Parental CHO-K1 cells.