INTRODUCTION
Bromodomain-contatining proteins are found in most tissues, including metabolic, nervous, reproductive, and connective tissues and serve important roles in many cellular processes (Park & Lee, 2020). Bromodomain containing protein 7 (BRD7) is a tumor suppressor gene associated with various cancers, including nasopharyngeal, breast, colorectal, prostate, lung, liver, uterine and ovarian cancers (Wu et al, 2009; Beroukhim et al., 2010; Wu et al., 2013; Chen et al., 2016; Gao et al., 2016; Yu et al., 2016). BRD7, also known as CELTIX-1, BP75, and NAG4, has a molecular weight of ~74 kDa, consists of 651 amino acids (Zhou et al., 2006; Liu et al., 2017), and forms part of the SW1/SNF complex. BRD7 expression is lower in several cancers, and its decreased expression is correlated with aggressive behavior in high-grade tumors. In ovarian cancer, BRD7 suppresses cancer growth independently of p53 and inhibits the beta (β)-catenin pathway by inhibiting its accumulation in the nucleus (Park et al., 2014). Recent studies have shown that the overexpression of BRD7 in ovarian cancer cells could inhibit cell invasion and viability as well as increase cell death (Park et al., 2012; Park et al., 2014). However, the critical role of BRD7 in cancers is still unknown.
The transforming growth factor β (TGF-β)-stimulated clone 22 (TSC-22) protein is induced by TGF-β in rat osteoblasts. TSC-22 has a molecular weight of ~17 kDa and consists of 114 amino acids (Shibanuma et al., 1992). It has a leucine zipper motif domain, which it uses to bind to a transcriptional regulator to inhibit transcription. Apoptosis is induced when TSC-22 is translocated into the nucleus (Kester et al., 1999; Hino et al., 2000). Decreased expression of TSC-22 has been observed in prostate cancer, salivary gland cancer, and brain tumors, and is associated with the growth and development of cancer cells (Nakashiro et al., 1998; Shostak et al., 2005; Rentsch et al., 2006; Nakamura et al., 2012). The overexpression of TSC-22 in ovarian cancer cells causes caspase-3-dependent apoptosis (Lee et al., 2008). In cervical cancer, TSC-22 interacts with the tumor suppressor p53, which prevents the proteasomal degradation of p53 and in turn prevents cell death (Yoon et al., 2012). Furthermore, TSC-22 binds to the oncogene colony stimulating factor-1 receptor and blocks the colony stimulation factor-1 (CSF-1) pathway (Cho et al., 2017).
Previously, we a performed yeast two-hybrid assay to screen a human ovary cDNA library for novel TSC-22 binding proteins and identified BRD7 as a candidate (data not shown). In this study, we investigated the interaction between TSC-22 and BRD7 and discovered a novel tumor suppression mechanism involving TSC-22 and BRD7.
MATERIALS AND METHODS
SKOV3 and OVCAR3 cell lines were obtained from the American Type Culture Collection. All cell lines were incubated at 37°C in a humidity 5% CO2 incubator, and cultured in each recommended medium (SKOV3, McCoy’s 5A Medium, OVCAR3, RPMI Medium) supplemented with 10% fetal bovine serum (Welgene, Gyeongsan, Korea) and 1% Amphotericin B/Streptomycin/Penicillin (Gibco, Grand Island, NY, USA). Cultures were replaced every 2–3 days, and all cells were cultured in 80%–90% confluence. Transfection was performed using the OmicsFect™ (CP2101) according to the manufacturer’s instructions.
BRD7 expression vector was constructed by cloning full length BRD7 into pcDNA3.1 vector using EcoR I and Xho I restriction enzymes. TSC-22 was cloned into the pcDNA3-flag vector using EcoR I and Xba I restriction enzymes. All restriction enzymes were purchased from Roche.
Cells were lysed in Pro-Prep (Intron, Seongnam, Korea) for 30 min on 4°C. The extracts were centrifuged at 13,000 rpm for 10 min at 4°C. Nuclear and cytoplasmic protein extraction was performed using the instructions of NE-PER Nuclear and Cytoplasmic Extraction Kit (Thermo Scientific, Waltham, MA, USA). All proteins were quantitated by using the Bradford assay. Protein samples were separated on 10%–12% SDS gels and transferred to nitro cellulose membranes. After blocking for 1 h at room temperature with 5% skim milk (Biopure, Cambridge, MA, USA), the membrane was incubated overnight at 4°C with primary antibody. After that, the appropriate secondary antibody was treated at room temperature for 1 h, and protein expression was confirmed using ChemiDoc™ XRS+ (Bio-Rad, Hercules, CA, USA). The antibodies used were as follows. TSC-22 (sc-101195), pERK (sc-514302), ERK (sc-154302), Elk-1 (sc-355), Egr-1 (sc-505830), GAPDH (25778), m-IgGκ BP-HRP (sc-516102), mouse anti-rabbit IgG-HRP (sc-2019) are purchased from Santa cruz and BRD7 (51009-2-AP) was purchased from Proteintech.
Transfected cells were lysed in NP-40 lysis buffer (20 mM Tris-HCl pH 8.0, 1% Nonident P-40, 150 mM NaCl, 1 mM PMSF) for 30 min on ice. Cell extracts were centrifuged at 13,000 rpm for 10 min at 4°C and obtained supernatant. All proteins were quantified using the Bradford assay. Each protein samples is reacted with TSC-22 antibody or BRD7 antibody for 16 h at 4°C, then immunoprecipitated with protein A/G PLUS agarose beads (sc-2003, Santa cruz Biotechnology, Dallas, TX, USA) for 4 h at 4°C. The immunoprecipitates was washed three times with lysis buffer and boiled in 1X SDS sample buffer for 5 min at 95°C. After centrifugation, the supernatant was analyzed by Western blot.
The cell growth was examined by the Cell Counting Kit-8 (CCK-8) assay. SKOV3 and OVCAR3 cells were seeded in 96-well plates and transiently transfected. CCK-8 reagent was treated and incubated for 1 h at 1 day intervals. The O.D value was measured at 450 nm in triplicate. To perform colony formation assay, SKOV3 and OVCAR3 were seeded 5×104–1.0×105 cells. After 24 h, cells were transiently transfected with plasmid vectors. After 24 h, the cells were counted and dispensed 1.0×103 cells in a 100 mm culture dish. The medium was changed every 3 days and cultured for 2 weeks to form colony. After the incubation, cells were washed twice with 1X PBS, fixed in 4% paraformaldehyde (Sigma-Aldrich, St. Louis, MO, USA) for 15 min and stained for 15 min at room temperature with 0.5% crystal violet (Sigma-Aldrich). The plates were rinsed with water and counted total colony number.
Each value represents the mean±SD of triplicate samples.
The EGY48 yeast strain was used in present study. BRD7 were cloned into pGilda vector using EcoRI and XhoI restriction sites and used as bait. TSC-22 was cloned into pB42AD vector using EcoRI and XhoI restriction sites and used as prey. Bait and prey vectors were co-transformed in EGY48 strain and the interactions were confirmed by cell growth assay on leucine-depleted yeast synthetic medium and by blue colony formation assay on X-gal containing medium. The binding activity between TSC-22 and BRD7 was determined by measuring the level of beta-galactosidase.
Cells were grown on 18 mm diameter cover glass (Paul Marienfeld GmbH & Co. KG, Lauda-Königshofen, Germany) and transiently transfected with the BRD7 or TSC-22 expression vectors. After 48 h incubation, the cells were rinsed twice with 1X PBS and fixed and permeabilized in methanol-acetone mixture (1:1) for 7 min at −20°C. Fixed cells were blocked with 5% BSA/PBS-T (PBS, 0.2% Tween-20) for 1 h at room temperature, and then incubated with BRD7 polyclonal antibody (1:300 dilution) and TSC-22 polyclonal antibody (1:300 dilution) for overnight at 4°C. The cells were washed three times with 1X PBS for 5 min each and incubated with alexa fluor 488 goat anti-rabbit IgG (Green) and alexa fluor 568 donkey anti-goat IgG (Red) antibody (Invitrogen, Carlsbad, CA, USA, 1,000:1 dilution) in darkness for 1 h at room temperature. Finally, the cells were counterstained with 1 μg/mL 4’,6-diamidino-2-phenylindole (DAPI) for 1 min at room temperature and mounted on slides. The signals and co-localization were detected using the confocal fluorescene microscopy.
RESULTS
To identify novel TSC-22-interacting proteins, yeast two-hybrid screening was performed using a human cDNA library, and we found that the BRD7 protein was a potential TSC-22 binding partner (data not shown). The potential interaction of TSC-22 with BRD7 was investigated using growth and β-galactosidase assays as part of a yeast two-hybrid system (Fig. 1A and B). To further examine the binding between TSC-22 and BRD7 in vitro, HEK293a cells were transfected with TSC-22 and BRD7 plasmids. Total cell lysates were immunoprecipitated with anti-BRD7 antibodies, then analyzed via Western blotting using specific anti-TSC-22 antibodies, which revealed a specific interaction between TSC-22 and BRD7 (Fig. 1C). We next assessed the subcellular localization of BRD7 and TSC-22 using immunocytochemistry. Fig. 1D shows that BRD7 and TSC-22 were co-localized mainly in the cytoplasm. Therefore, these results suggest that BRD7 and TSC-22 strongly interact with each other in the cytoplasm.
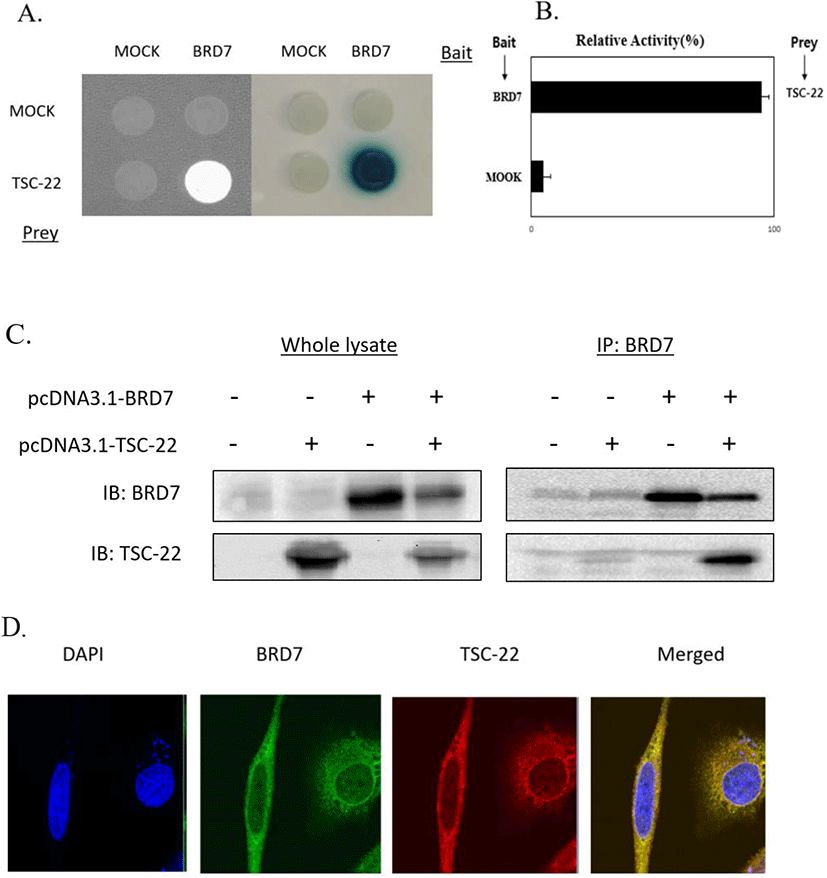
We next explored the effects of TSC-22 and BRD7 on ovarian cancer cell growth by transfecting SKOV3 and OVCAR3 cancer cells with TSC-22 and/or BRD7 expression vectors and monitoring cell growth for 24-72 h. As shown in Fig. 2A, the overexpression of TSC-22 and BRD7 inhibited cell growth by 25% and 20% in SKOV3 cells and by 25% and 30% in OVCAR3 cells, respectively. Following transfection with both TSC-22 and BRD7, tumor cell growth was reduced by 50% in SKOV3 cells and OVCAR3 cells compared to the control. These results suggest that TSC-22 and BRD7 work synergistically to inhibit tumor growth.
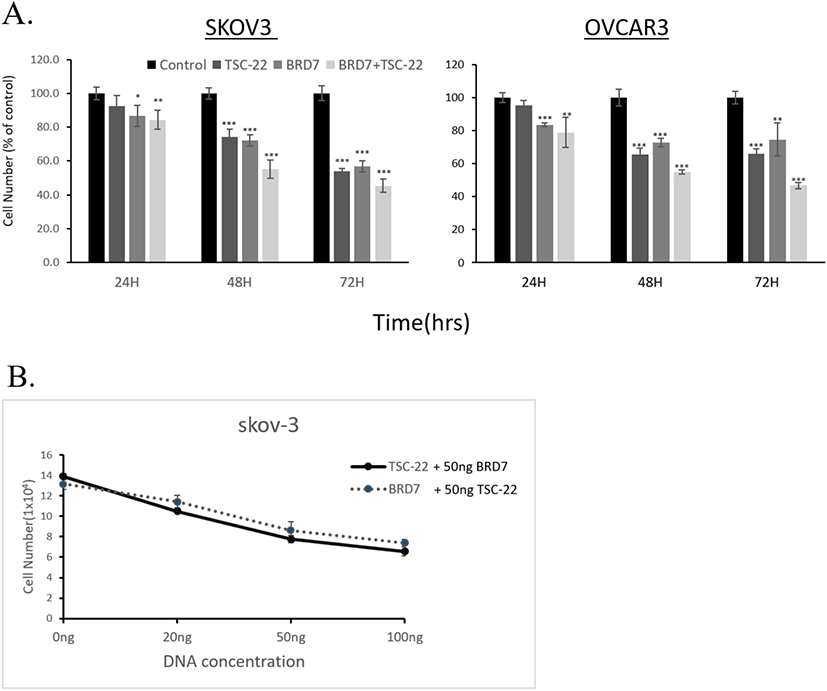
To further investigate the functional relationship between TSC-22 and BRD7, cells were co-transfected with 50 ng of the BRD7 expression vector and various amounts (20, 50, 100 ng) of the TSC-22 expression vector. This experiment was repeated with cells co-transfected with 50 ng of TSC-22 expression vector and varying amounts (20, 50, 100 ng) of the BRD7 expression vector. We found that the combined transfection of TSC-22 and BRD7 significantly inhibited cell growth in a dose-dependent manner (Fig. 2B). We then compared the ability of each transfected cell line to form colonies. Consistent with the cell growth data, SKOV3 cells transfected with TSC-22- or BRD7-expressing vectors formed significantly fewer colonies compared to the control vector-transfected cells. The co-transfection of TSC-22 and BRD7 significantly reduced colony formation in SKOV3 (Fig. 3). Our results suggest that the interaction between TSC-22 and BRD7 significantly reduces cancer cell proliferation.
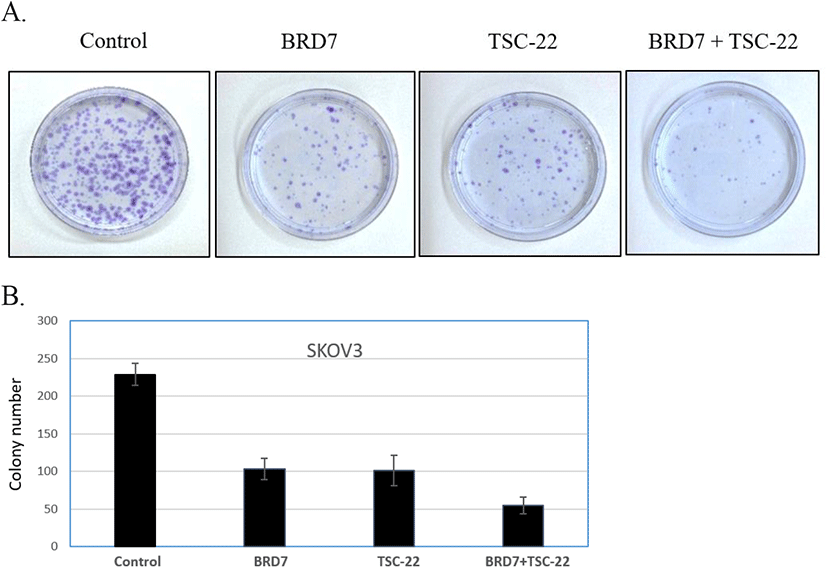
Next, we investigated the effects of the TSC-22 and BRD7 interaction on the ERK signaling pathway. SKOV3 and OVCAR3 cells were transfected with TSC-22 and/or BRD7. Total protein extracts were subjected to a Western blot assay using antibodies specific for several proteins that mediate the ERK signaling pathway. Following co-transfection with TSC-22 and BRD7, the phosphorylation of ERK and its downstream signaling proteins Elk-1 and Egr-1 were reduced and showed synergic inhibition effect (Fig. 4A and B).
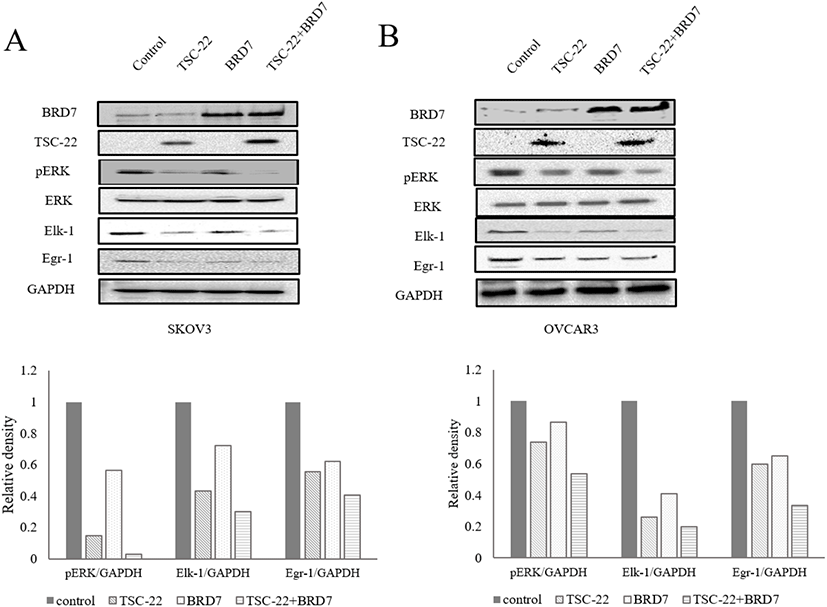
Our results suggest that the interaction between TSC-22 and BRD7 enhances the activity of both proteins. Together, our results show that the interaction between TSC-22 and BRD7 inhibits the ERK pathway thus suppressing tumor cell growth.
DISCUSSION
In this study, we discovered a critical function of TSC-22 that it is an interaction partner and synergic regulator of BRD7, and this interaction may have an impact on ovarian cancer. Here, we suggest a possible mechanism for the inhibition of tumor growth and proliferation by TSC-22 and BRD7 involving their direct binding, which triggers the inhibition of the ERK signaling pathway and prevents the translocation of β-catenin from the cytoplasm to the nucleus.
Ovarian cancer has no early symptoms, so most patients receive their first diagnosis at stage 3. Therapies for ovarian cancer, such as surgery, chemotherapy, and radiation therapy, are limited. Therefore, research into the anti-cancer mechanisms of tumor suppressor genes is being actively pursued to identify new targets and treatments for ovarian cancer. TSC-22 interacts with various oncogenes and tumor suppressor genes and inhibits the growth and development of cancerous cells (Yoon et al., 2012; Cho et al., 2017). However, the mechanism behind the tumor suppression activity of TSC-22 is still unknown.
We therefore screened for TSC-22-binding proteins and identified BRD7 as an interaction partner, which we used to probe the anti-cancer mechanisms of TSC-22 (Fig. 1).
BRD7 has low expression levels in many cancers, where it inhibits cancer cell growth and triggers apoptosis (Kikuchi et al., 2009; Drost et al., 2010; Harte et al., 2010). BRD7 regulates cell growth and proliferation via the ERK and β-catenin pathways. The β-catenin pathway, which is downstream of the Wnt signaling pathway, is associated with cancer. Translocation of β-catenin from the cytoplasm to the nucleus promotes the development of tumors in the breast, rectum, prostate, lung, esophagus, and ovary (Taketo, 2004; Anastas & Moon, 2013). The levels of both TSC-22 and BRD7 are also low in ovarian cancer cells (Yu et al., 2016).
Our results showed that the overexpression of TSC-22 and BRD7 in two typical ovarian cancer cell lines, SKOV3 and OVCAR3, reduced cell growth (Fig. 2). Our work also suggested that the direct binding of TSC-22 and BRD7 inhibited the growth of cancer cells. We found that the inhibitory effects of TSC-22 and BRD were more pronounced when the two proteins were overexpressed together in the same cell line compared to when they were overexpressed individually (Figs. 2 and 3). When TSC-22 and BRD7 were expressed individually, cancer cell growth rates were lower compared to that in the control; however, this effect was amplified when TSC-22 and BRD7 were co-expressed, suggesting functional synergy between the two proteins.
In this study, we investigated how BRD7 and TSC-22 inhibit the growth and proliferation of ovarian cancer cells. We found that the interaction between TSC-22 and BRD7 inhibited the ERK pathway (Fig. 4). BRD7 is involved in the β-catenin and ERK pathways (Park et al., 2012; Gao et al., 2016). We found that the inhibitory effect was stronger in cells co-expressing BRD7 and TSC-22, compared to cells overexpressing BRD7 only. The dysregulation of the β-catenin and ERK pathways promotes transformation to cancer in several types of cancer cell (Yang et al., 2008; Shang et al., 2017). This suggests that the tumor suppressor effects of TSC-22 and BRD7 that we observed in ovarian cancer cells could potentially be translatable to other cancer types.
The inhibitory effects on cancer cell growth driven by the BRD7/TSC-22 interaction differed between the SKOV3 and OVCAR3 cell lines. Our cell growth assay and Western blots revealed that the inhibitory effects of BRD7 and TSC-22 co-overexpression were greater in SKOV3 cells than in OVCAR3 cells. This suggests that the synergistic effects of TSC-22 and BRD7 may manifest differently in SKOV3 and OVCAR3 cells. We hypothesize that these differences may be associated with p53. Both TSC-22 and BRD7 bind to p53 (Drost et al., 2010; Yoon et al., 2012); TSC-22 binds to p53 to protect it from degradation, and binding between p53 and BRD7 enables the localization of BRD7 to p53-regulated promoters (Yoon et al., 2006; Mantovani et al., 2010). p53 is a principal tumor suppressor gene and exhibits decreased expression or altered function in most cancer types (Meek, 2015). The SKOV3 ovarian cancer model lacks p53, whereas OVCAR3 cells have a mutant version of p53 (Park et al., 2012). We therefore hypothesize that the presence or absence of the p53 protein in the cancer cell lines caused differences in the synergic effect from the BRD7 and TSC-22 interaction.
The tumor suppressor activity of BRD7 has been observed in several carcinomas, where it inhibited the Ras/MEK/ERK and Wnt/β-catenin pathways. The ERK pathway is associated with various functions such as cancer proliferation and cell survival, differentiation, and migration, and is aberrantly activated in many human cancers. Thus, blocking the ERK pathway is an attractive target for anti-cancer therapeutics.
In a previous study, we found that TSC-22 inhibited the ERK pathway by inhibiting the CSF-1 pathway (Cho et al., 2017). Here, we discovered that TSC-22 potentiated the ERK-inhibitory activity of BRD7. Our results suggest that TSC-22 is a promising cancer treatment target, as it can inhibit ERK activity via various pathways. Our study revealed the synergistic, anti-cancer effects of TSC-22 and BRD7 in ovarian cancer cells, thus opening the door to new cancer treatment possibilities.