INTRODUCTION
Morphological differences in groups and species are classified in terms of the overall body shape or specific morphoanatomical characteristics (Sträuss & Bond, 1990). Among the body shape characteristics of fish, morphometric traits, unlike meristic traits, can be measured as mensural characteristics. Although the body shape of fish is largely determined by genetic factors (Riddell et al., 1981; Taylor & McPhail, 1985), the use of morphometric analyses to distinguish genetically discrete groups within a fish species is limited by the difficulty of measuring environmentally induced variations in body shape (Ihssen et al., 1981; Currens et al., 1989; Park et al., 2001).
Both truss and classical dimensions are used to describe fish body shapes (Hubbs & Lagler, 1947; Sträuss & Bookstein, 1982). Truss dimensions consist of a systematically arranged set of distances that are measured between a set of preselected anatomical landmarks (Sträuss & Bookstein, 1982; Sträuss & Bond, 1990). These landmarks are identified based on local morphological features and are chosen to divide the body into functional units (Sträuss & Bond, 1990). Truss dimensions, which include components of body depth and length along the longitudinal axis, have theoretical advantages over classical morphometric characteristics in discriminating different groups (Park et al., 2007).
The cyprinid loach, Misgurnus anguillicaudatus, is a freshwater fish species of the loach family Cobitidae (Nelson, 2006). The species is native to East Asia, but is also popular as an aquarium fish and has been introduced elsewhere in Asia and to Europe and North America (Park et al., 2006c). The cyprinid loach inhabits mud, ponds, and rice fields, which are subject to periodic drying (Park & Kim, 2000), and this can result in starvation. The domestic market for this species has expanded rapidly in recent years, and consequently, the cyprinid loach is now a commercially important freshwater species in Korea (Park et al., 2006c). Therefore, it is cultivated in Korea with high marketability. However, our knowledge of the early larval development of the cyprinid loach is limited.
The visual function of fish larvae is very important for feeding, positioning, collective behavior, and escape from predators (Rodriguez & Gisbert, 2001; Park et al., 2006a). The retinal structure of the Teleostei is not distinct from the retinal structure of vertebrates. However, although it is comparable to that of other vertebrates, it is difficult to propose a general retinal structure for the Teleostei because of the many species, various habitat, numerous activities, and different life cycle in this taxon (Wagner, 1990). The structure and function of the visual apparatus, the behavior of the organism, and environmental influences are very important factors in visual ecology (Walls, 1942; Lythgoe, 1979).
It is well recognized that to successfully rear larval fish, the culture conditions, feeding strategies, and survival rates must be considered in terms of the ontogenetic status of the larvae. The early survival rates of marine fish are influenced by the success or failure of the first feeding, with the transition from endogenous feeding to exogenous feeding after the reduction of the yolk sac (O’Connell, 1976; Theilacker, 1978; Strussmann & Takshima, 1990). Thus, it is important to understand the development of the alimentary tract tissues, organogenesis, and the time at which the yolk sac is completely absorbed during growth to increase the early survival rate of fish.
More information about the developmental stages of the cyprinid loach is required because high levels of hatchery mortality are common during the early life stages of reared larvae. However, there have been no detailed reports of the anatomy of this species other than those describing the morphological and histological changes that occur in cyprinid loach larvae (Park & Kim, 2000; Park et al., 2006b). Because research into the early larval development of the cyprinid loach is currently insufficient, in this study, we investigated the process of yolk absorption in M. anguillicaudatus, determined the changes in its morphometric characteristics, and observed the histological changes that occur in the retina, kidney, and midgut epithelium.
MATERIALS AND METHODS
We used larval specimens of the cyprinid loach, M. anguillicaudatus (n=30) that hatched on June 28, 2012. We obtained fertilized eggs from Southern Island Fishery Research Institute of the National Fisheries Research and Development Institute (NFRDI) in Korea. They were reared and bred in the Laboratory for Fisheries Genetics and Breeding Sciences, Korea Maritime and Ocean University, Busan, Korea, in three tanks (100 ℓ) that included a circulation pump, an aeration system, and a temperature-control system. Dissolved oxygen levels were maintained with an air pump and the water temperature was maintained at 26±0.5°C. Standard length of female and male were 15.6±1.88 cm and 16.1±1.42 cm, respectively. Body weight of female and male were 10.2±0.43 g and 10.4±0.49 g, respectively.
To measure the eye diameter, yolk length (YL), yolk height (YH), and yolk volume (YV), we fixed 50 larval cyprinid loaches, ranging in age from just hatched to 16 days posthatching (DPH), in 10% neutral formalin for two days. We also measured the larvae of the cyprinid loach in the yolk stage to the nearest 1 mm, using the scale and Vernier calipers (CD-20CP; Mitutoyo, Japan). We measured YL and YH according to number of days after hatching; and YV was measured with the mathematical formula YV = (π/2) × a × b2, where a is yolk length, b is yolk height (Blaxter & Hempel, 1963).
To determine the morphometric characteristics of the cyprinid loach, 30 samples were randomly selected at 10-day intervals from 20 DPH to 60 DPH and fixed in 10% neutral formalin. Body outline measurements were made for 25 distances between the landmarks used for both truss and classical dimensions (Table 1, Fig. 1). We measured the larvae each day to the nearest 1 mm using the scale and Vernier calipers, and their weights were measured with an electronic balance. The horizontal distance measurements made were: Ls, standard length; HHAV, horizontal distance between the most anterior extension of the head and the anterior insertion of the ventral fin; HHAP, horizontal distance between the most anterior extension of the head and the anterior insertion of the pectoral fin; HDAC, horizontal distance between the most anterior extension of the anal fin and the anterior insertion of the caudal fin; and ED, eye diameter. The other distances (truss morphometric dimensions and classical morphometric dimensions) were determined from the direct distance measurements.
![]() |
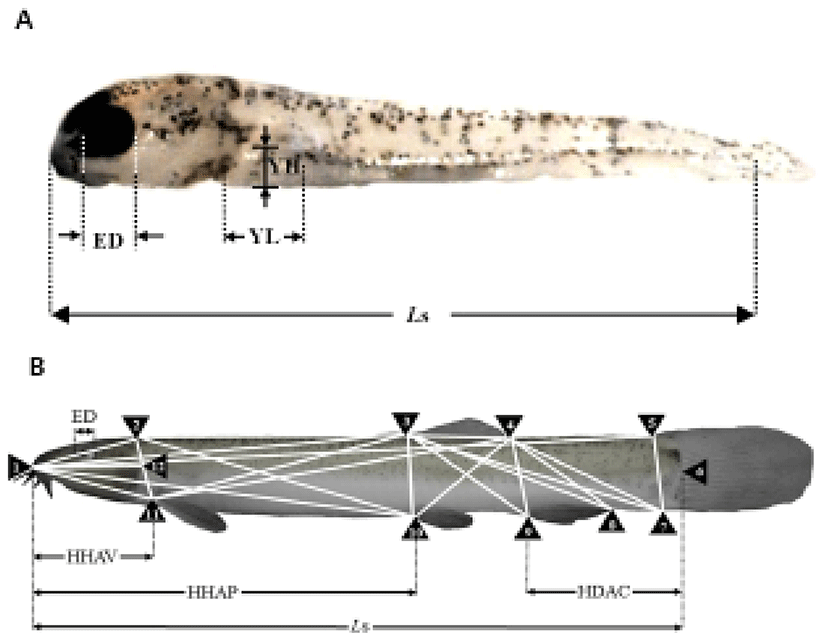
The external truss morphometric dimensions were 2 × 3, the posterior end of the supraoccipital × the origin of the dorsal fin; 2 × 10, the posterior end of the supraoccipital × the origin of the pelvic fin; 2 × 11, the posterior end of the supraoccipital × the origin of the pectoral fin; 3 × 4, the origin of the dorsal fin × the insertion of the dorsal fin; 3 × 7, the origin of the dorsal fin × the ventral origin of the caudal fin; 3 × 8, the origin of the dorsal fin × the insertion of the anal fin; 3 × 9, the origin of the dorsal fin × the origin of the anal fin; 3 × 10, the origin of the dorsal fin × the origin of the pelvic fin; 3 × 11, the origin of the dorsal fin × the origin of the pectoral fin; 4 × 7, the insertion of the dorsal fin × the ventral origin of the caudal fin; 4 × 8, the insertion of the dorsal fin × the insertion of the anal fin; 4 × 9, the insertion of the dorsal fin × the origin of the anal fin; 4 × 10, the insertion of the dorsal fin × the origin of the pelvic fin; 4 × 11, the insertion of the dorsal fin × the origin of the pectoral fin; and 5 × 7, the dorsal origin of the caudal fin × the ventral origin of the caudal fin (Fig. 1). Each external morphometric dimension was analyzed relative to Ls.
The external classical morphometric dimensions were: 1 × 2, the most anterior extension of the head × the posterior end of the supraoccipital; 1 × 3, the most anterior extension of the head × the origin of the dorsal fin; 1 × 5, the most anterior extension of the head × the origin of the caudal fin; 1 × 9, the most anterior extension of the head × the origin of the anal fin; 1 × 10, the most anterior extension of the head × the origin of the pelvic fin; 1 × 11, the most anterior extension of the head × the origin of the pectoral fin; and 1 × 12, the most anterior extension of the head × the most posterior aspect of the operculum (Fig. 1). Each external morphometric dimension was analyzed relative to Ls.
To observe the organogenesis of the cyprinid loach, 30 samples were randomly selected at 10-day intervals from just hatched to 50 DPH and fixed in 10% neutral formalin. We extracted the eye, kidney, and midgut epithelium from the fish sampled at just hatched, 10 DPH, 30 DPH, and 50 DPH for histological analysis. Each sample was fixed in Bouin’s solution for 24 h and then washed in flowing water. To decalcify the samples, they were processed in decalcification solution for 24 h and washed again. The samples were then dehydrated in a graded series of alcohol: successively in 70%, 80%, 90%, and 100% alcohol for 1 h each. After they were cleared with xylene for impregnation, the samples were treated with soft paraffin and hard paraffin, embedded, trimmed, and sectioned. The sections were then stained with hematoxylineosin and mounted with Canadian balsam. After we had determined the critical points of the samples, we photographed them with an optical microscope equipped with a camera (Axiocam MR, Carl Zeiss, Germany).
Using an eyepiece micrometer under an optical microscope (Axiocam MR), we used the methods of Park et al. (2006c) to measure the thickness of the retina; the epithelial layer (EL), the rod and cone layer (RCL), the outer limiting membrane layer (OLM), the inner nuclear layer (INL), the inner plexiform layer (IPL), and the ganglion cell layer (GCL). We also photographed the eye structure of the larvae from just-hatched to 50 DPH using an optical microscope. To analyze the development of the kidney and midgut epithelium, we used the Axioskop 4.1 image analysis software (Carl Zeiss, Germany) to measure the areas and volumes of the cells and nuclei using the following formulae: surface area = 1/4 × abπ, and volume = 4/3 × π(a/2) × (b/2)2, where a is the major axis of the cell or nucleus; and b is the minor axis of the cell or nucleus (Park & Kim, 2000).
The experiment was performed in triplicate and the results are reported as means ± SD (n = 30), unless otherwise stated. The data were analyzed with one-way ANOVA using the SPSS statistical package (SPSS 9.0, SPSS Inc., Chicago, IL, USA). Means were compared using Duncan’s multiple range test and were considered significantly different at P<0.05.
RESULTS
Table 2 shows the one morphometric dimension and three morphometric dimensions/Ls of the cyprinid loach, Misgurnus anguillicaudatus, ranging in age from just hatched to 16 DPH. At 1 DPH, the eye was 30.4. Thereafter, the eye ratio decreased to 7.8 at 16 DPH. YL also decreased from 18.4 to 2.7 at 16 DPH. YH gradually decreased from 7.7 to 1.7 at 16 DPH (P<0.05). During the experimental period from 1 DPH to 16 DPH, YL was always greater than YH (P<0.05). YV decreased most rapidly of all the values to 16 DPH.
![]() |
Figures 2 and 3 show growth curves for the cyprinid loach. The increase in Ls was y = 0.1x + 0.48, R2 = 0.8777 (y is standard length in mm, x is DPH); the increase in body weight was y = 10.9x–18.80, R2 = 0.8552 (y is body weight in mg, x is DPH). The increases in standard length and body weight showed a positive relationship. Standard length increased to 0.7 mm from 30 DPH to 50 DPH. Thereafter, the cyprinid loach grew to 2.27 mm (Fig. 2) and its body weight increased to 90.2 mg at a steady rate to 60 DPH (Fig. 2). The rate of increase in body weight was more regular than the increase in standard length (Fig. 2). The body weight/standard length relationship was y = 38.8x – 16.79, R2 = 0.8478 (y is body weight in mg, x is standard length in mm) (Fig. 3). Body weight and standard length increased proportionally (Fig. 3). Although the average body weight/standard length ratio was 38.8, body weight/standard length in the point of standard length 0.65 –1 mm was 14.28, and 1.25–1.55 mm was 20, which indicates a slower increase in body weight than in standard length (Fig. 3).
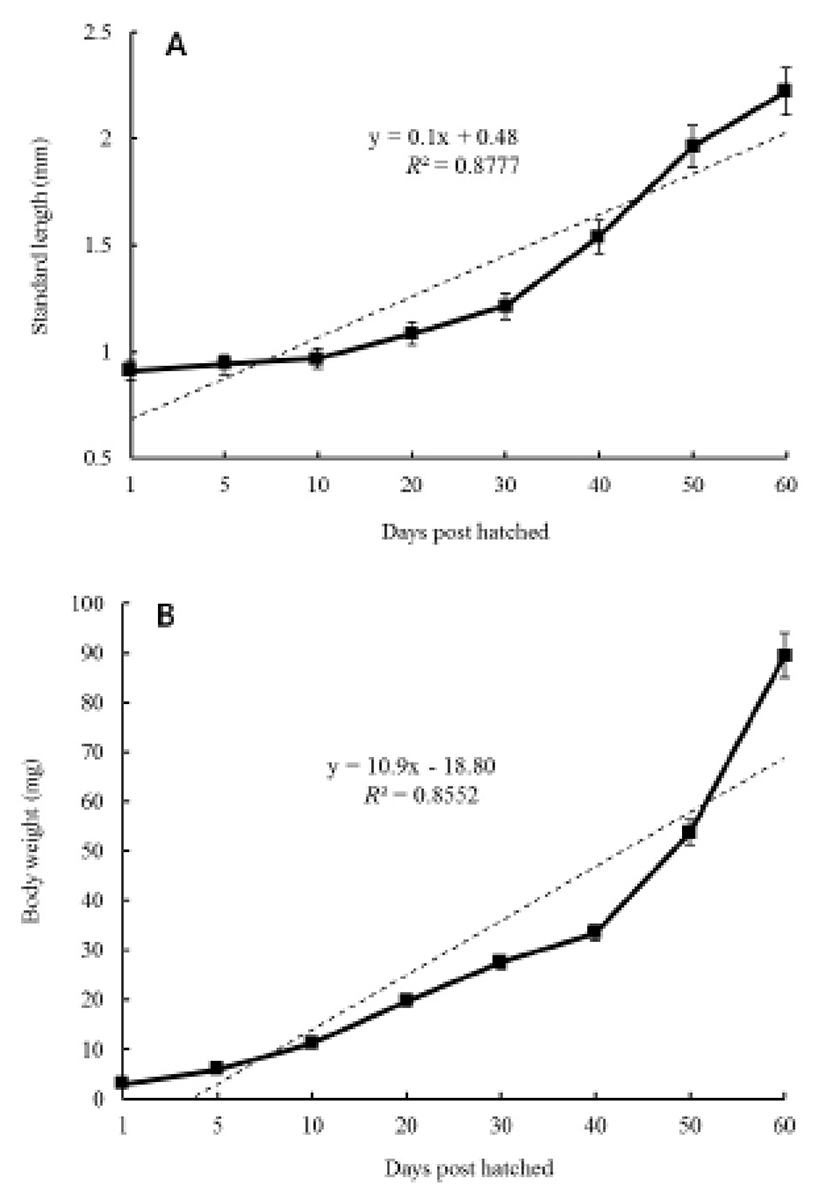
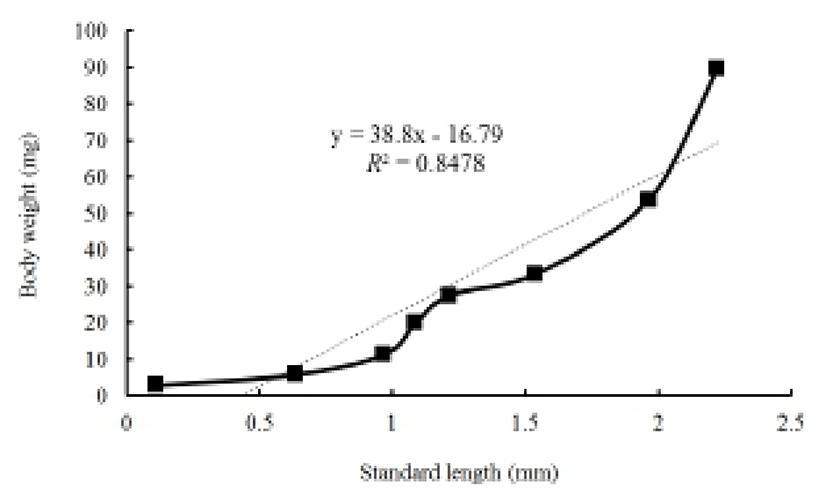
Table 3 show 26 morphometric dimensions/Ls of the cyprinid loach, measured every 10 days from 20 DPH to 60 DPH: 2 × 11, 4 × 8, and 4 × 9 did not differ significantly from 30 DPH to 60 DPH (P>0.05), and 3 × 4, 3 × 8, and 3 × 10 did not differ significantly from 40 DPH to 60 DPH. During the 60 DPH, 1 × 2, 1 × 3, 1 × 11, 2 × 10, and 3 × 7 decreased gradually (P>0.05), whereas 1 × 5, 3 × 9, 3 × 11, and 4 × 10 increased gradually (P<0.05); 3 × 11 and 2 × 3 increased by 11% and 9%, respectively, and this was the greatest growth rate in the external characteristics of the fish, occurring in a section of fin (P<0.05). As shown in Fig. 4, the patterns of increase in the morphometric characteristics measured in this study can be classified in three ways. HHAV, 1 × 3, and 1 × 9 are the main traits of each curve (Fig. 4). In the point of standard length 1.78 mm, the length of each dimensions of 1 × 3 series were only changed. At the same point, the other series of curves gradually increased (Fig. 4).
![]() |
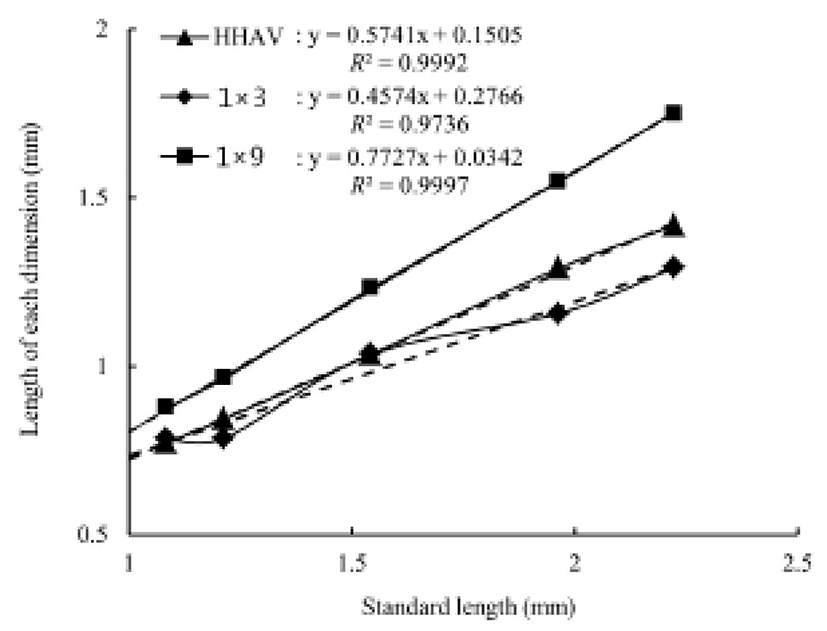
Table 4 shows the component layers of the retina in the cyprinid loach from just hatched to 50 DPH. The thickness of the retina decreased from just hatched to 10 DPH, but increased continuously thereafter to 50 DPH (P<0.05). The thickness of the RCL increased most rapidly, whereas GCL decreased most dramatically of all the component layers over 50 days (P<0.05). Table 5 shows the cell sizes of the proximal tubule in the kidney and the nuclear height of the cells of the midgut epithelium in the fish from hatching to 50 DPH. The nuclear height of the midgut epithelium increased continuously from just hatched to 50 DPH (P<0.05). The major and minor axes of the proximal tubule cells in the kidney increased with time, and the minor axes of the proximal tubule cells were shorter than the major axes (P<0.05). The surface area and volume of the proximal tubule cells in the kidney increased dramatically with time (P<0.05).
![]() |
![]() |
Figures 5 and 6 shows the histological changes in the eye, kidney, and midgut epithelium of the cyprinid loach from just hatched to 50 DPH. The post-hatching eye shows a much looser layer structure than that in the eye at 50 DPH (Fig. 5). The RCL structure is particularly loose in the just-hatched eye, although it is densely concentrated at 50 DPH. The kidney consists of many proximal tubules (PT), appearing as hollow ovals and circles. At 50 DPH (Fig. 6B), the kidney has more and larger PT than the just-hatched kidney (Fig. 6A). The greatest change in the three tissues examined occurred in the midgut epithelium during the 50 days of the experiment. The midgut epithelium in the just-hatched larvae (Fig. 6C) is stretched and straight, whereas at 50 DPH (Fig. 6D), it is wrinkled and folded, and the tissue has also become very dense (Fig. 6D).
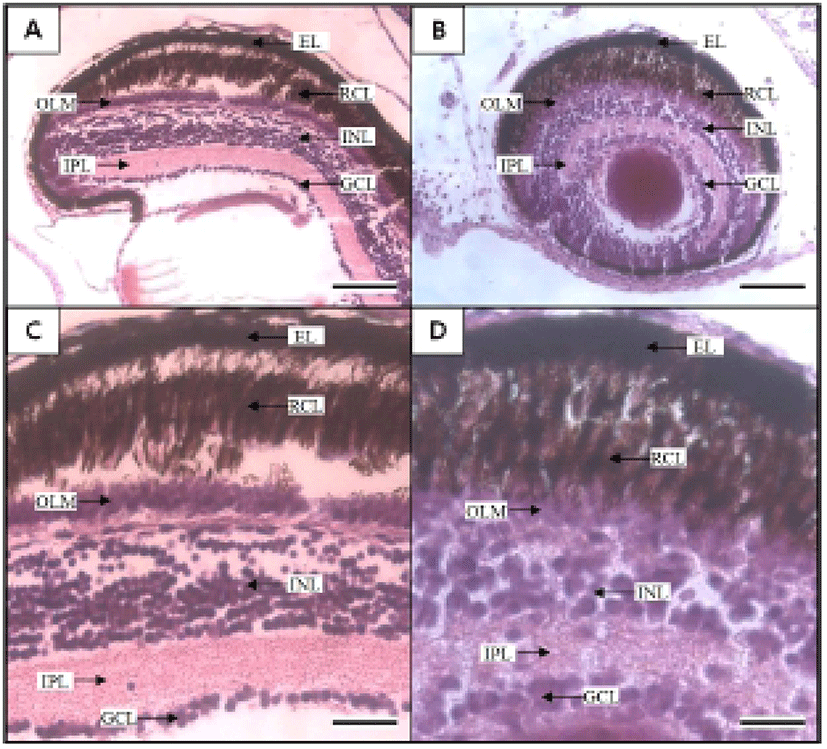
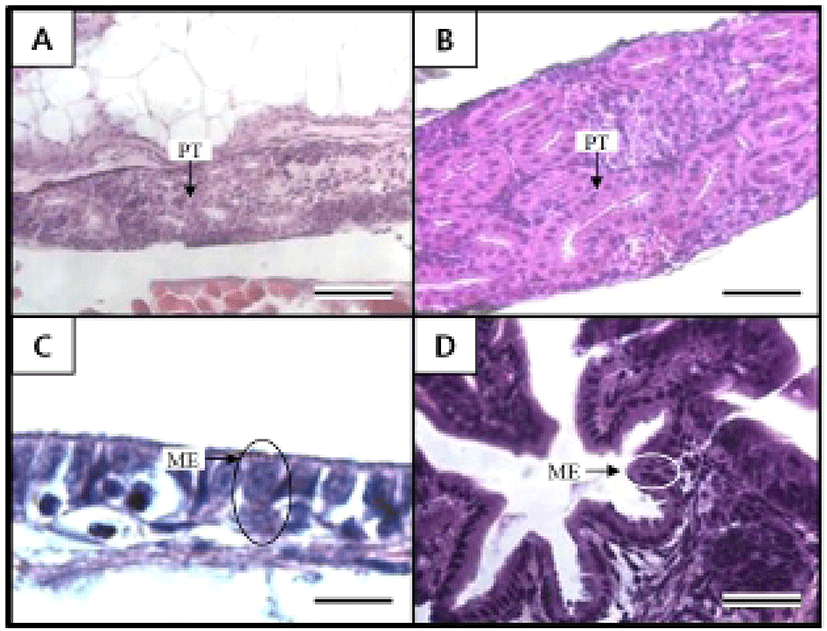
DISCUSSION
The truss dimensions are the primary units of classification for the external anatomical markers that are normally assessed in fish (Sträuss & Bond, 1990). The truss dimensions are length measurements that refer to the ratios between the width of the fish’s body and the axis of the fish’s length. Theoretically, the truss dimensions are a better measurement of the fish’s shape than the classical dimensions (Sträuss & Bookstein, 1982; Currens et al., 1989).
The combined morphometric traits of the cyprinid loach, Misgurnus anguillicaudatus, showed a tendency to increase from 20 DPH to 60 DPH (Table 3). However, 2 × 10 and 3 × 10 in the abdominal section showed some reduction with the absorption of the yolk sac. In this study, the day upon which the yolk sac was completely absorbed coincided with the reduction in 2 × 10 and 3 × 10 in the abdominal section. HHAV, HHAA, and HDMC increased during the experimental period (Table 3), which suggests that after hatching, the body length increased with time, although the shape and ratios described by HHAV, HHAA, and HDMC did not change significantly (P>0.05). Consequently, during the early growth of the cyprinid loach, the horizontal dimensions decreased significantly.
In research related to the early growth of M. anguillicaudatus, it has been unclear when the whole yolk sac is absorbed and the larva begins to emerge, and there has been no information on how the shape of the yolk sac changes as it decreases in size as it is absorbed. In this study, YL decreased with time. After hatching, YH also decreased but the decrement was smaller than that of YL. We found that the decrement in YL was greater than that in YH. Consistent with the reductions in YL and YH, YV also declined, and the decrement in YV was the greatest among the three measures. This reduction in YV means that like alevin, the endogenous feeding of the juvenile is diverted to exogenous feeding as the yolk sac diminishes (Zhang et al., 1955; Dill, 1969, 1977; Brannon, 1972).
In the Teleostei, the eye usually continues to grow in the adult period, but the optic characteristics of the eye component stabilize in the early days of development. The eye of the cyprinid loach was completely formed after hatching. Its retina is composed of six layers (EL, INL, INL, IPL, OLM, and RCL), and each layer shows similar characteristic to those of other cyprinid fish (Takashi, 1982) (Fig. 5A, 5B). In this study, OLM in the cyprinid loach did not change significantly (P>0.05), but there was a significant change in RCL (P<0.05). This result is consistent with the study of Park & Kim (2000). Similar research has also been conducted in the dark-banded rockfish, Sebastes intermis, and reported that unlike the cyprinid loach, RCL in the dark-banded rockfish does not change significantly during development (P>0.05), although there is a significant change in OLM (P<0.05) (Park et al., 2012). These changes are completely opposite those observed in the cyprinid loach. Based on these results and the intrinsic functions of the retinal components, we infer that the rates of change in the retinal components of a fish are related to the physiological ecology of the fish in the early days of its development.
The early development of the internal organs is consistent across most Teleostei (Blaxter, 1988). Generally, the kidney displays one of two types of developmental processes, forming either after hatching or before hatching. In this study, the kidney and PT were already formed when the fish hatched and developed completely during the first 50 DPH. This also occurs in the dentex, Dentex dentex, and like the cyprinid loach, the kidney of dentex is already formed when the fish hatches and grows continuously after hatching (Santamaria et al., 2004). As mentioned by Park & Kim (2000), the major axes of the proximal tubule cells in the kidney of the cyprinid loach is 39.06 ± 2.98 μm, and the minor axes and surface area are 31.38 ± 2.04 μm and 954.7 ± 2.98 μm, respectively. In the previous study, the cyprinid loach was examined 10 months after hatching, whereas the samples in our study were examined at 50 DPH. Therefore, the combined results of Park and Kim (2000) and this study suggest that the kidney of the cyprinid loach continues to develop after 50 DPH.
It is generally reported that the absorptive function of the alimentary canal in fish is initiated either before or after the absorption of the yolk sac. In juvenile Coregonus fera, the yolk sac is completely absorbed after 11 DPH, and during this period, the absorptive function of the midgut epithelium begins to function (Loewe & Eckmann, 1988). In the juvenile summer flounder, Paralichthys dentatus, the yolk sac is completely absorbed after 3 DPH, and at 4 DPH, the absorptive function of the midgut epithelium begins to function (Bisbal & Bengtson, 1995). However, in the juvenile pike eel, Muraenesox cinereus, the absorptive function of the midgut epithelium begins at 3 DPH, before the complete absorption of the yolk sac. At this time, although the mucosal fold in the midgut is not formed, microvilli structures can be detected in the midgut with optical microscopy and the active absorption of horseradish peroxidase was detected in these cells. Thus, liquefactive nutriments are presumed to be absorbed at this time (Otake et al., 1995). The midgut of the cyprinid loach is already present when it hatches and grows gradually to 50 DPH. Because the yolk sac in this fish is completely absorbed by 14 DPH, the absorptive function of the midgut is thought to begin before the yolk sac is completely absorbed, as in the pike eel.
As mentioned above, there has been insufficient research into the early growth of the cyprinid loach. In this study, the histological changes, morphometric characteristics, and absorption of the yolk sac were investigated during the experimental period from 1 DPH to 60 DPH. Studies that correlate the different aspects of the early growth of the cyprinid loach, based on the results of this research, must be conducted.