Increase of intracellular free calcium concentration ([Ca2+]i) is monitored in various cellular response to stimuli (Berridge et al., 2000). [Ca2+]i increases is observed in most of cellular response such as secretion and contraction, proliferation, differentiation, fertilization, development and cell death (Berridge et al., 2003). Fertilization of mammalian oocytes, one of the cellular event, is controlled by increase in [Ca2+]i with oscillation pattern that persists for several hours from sperm-egg fusion (Miyazaki et al., 1993). These [Ca2+]i oscillations are responsible for the initiation of fertilization event including, cortical granule exocytosis to block to polyspermy, resumption of meiosis, recruitment of maternal mRNAs and activation of embryonic genome, and further embryonic development (Ajduk et al., 2008). Most Ca2+ -increase during fertilization originates from intracellular calcium store via 1, 4, 5 inositol triphosphate (IP3) receptors (IP3R) on the endoplasmic reticulum membrane. IP3 is a widespread signaling molecule produced as a result of hydrolysis of phosphatidylinositol 4, 5-bisphosphate (PIP2) by phospholipase C (PLC) (Lee et al., 2006b). Egg has reported to express all three isoforms of IP3R, but type 1 IP3R (IP3R1) is present significantly larger amount in mammalian eggs (He et al., 1999). This review is to introduce the general information of IP3R and role of IP3R in mammalian egg fertilization.
[Ca2+]i OSCILLATION DURING FERTILIZATION
Fertilization is a process which is regulated by IP3-mediated Ca2+-release from intracellular Ca2+-store. In mammalian oocytes, the sperm factor (SF, now it has been as known phospholipase C zeta, PLCζ) triggers activation of phosphoinositide (PI) pathway that produces IP3 and 1, 2-diacylglycerol (DAG) by hydrolysis of PIP2. Increase intracellular IP3 concentration is in charge for mediating Ca2+-release from endoplasmic reticulum (ER) via IP3 receptor (Fig. 1) (Miyazaki et al., 1993; Malcuit et al., 2005; Malcuit et al., 2006; Yoon, 2011). Microinjection of 18A10 antibody, which recognizes an epitope to the Ca2+ channel region in the C-terminus of IP3R1, or IP3R antagonist inhibits [Ca2+]i oscillation in fertilized oocytes (Miyazaki et al., 1992). Also, adenophostin A, agonist of IP3R, or thimerosal, a thiol oxidizing agent, induced [Ca2+]i oscillation and further egg activation process in mature oocytes without sperm (Fissore et al., 1992; Jellerette et al., 2000).
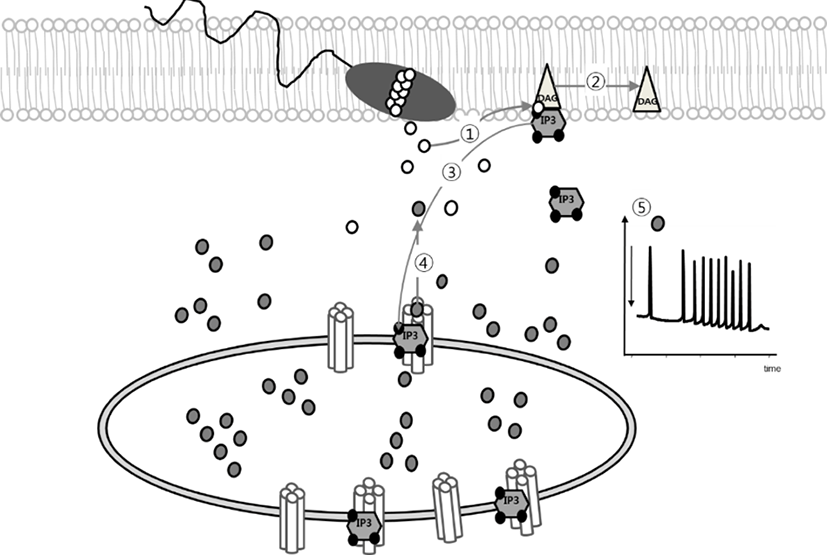
GENERAL STRUCTURE OF IP3R1
IP3, as an intracellular second messenger, mediating Ca2+ -signalling in cellular response was first reported in neutrophils (Prentki et al., 1984). Autoradiographic studies with [3H] IP3 represented high densities of IP3 binding in cerebellum with 100-300 times higher than in peripheral tissues (Worley et al., 1987). The purification of IP3R was reported 260 KDa protein band by electrophoretic analysis, purified protein presented seletivity for IP3 over other inositol phosphates (Supattapone et al., 1988). Three isoforms of IP3R (type 1, type 2, and type 3) have been identified by cDNA cloning. Most cells express at least one isoform and type 1 IP3R (IP3R1) is the most widely expressed in all cell types and all developmental stages (Marks, 1997).
The three IP3R isoforms demonstrate different IP3-binding affinities and sencitivity. The overall domain structure of IP3R contains a cytoplasmic N-terminus, IP3 binding domain, regulatory or modulatory domain which has multiple regulatory sites for Ca2+, calmodulin dependent protein kinase, and other regulatory protein (Fig. 2). The C-terminus is channel domain including six transmembrane domains (TMD), which form Ca2+-release channels on the endoplasmic reticulum membrane (Lee et al., 2006b).
Using electron microscopy analysis, IP3R was observed tetrameric structure with reversible transition between two distinct structure, windmill and square structure (Hamada et al., 2002).
ACTIVATION OF IP3R
Ca2+-release through IP3R is known to be modulated by posttranslational modification of IP3R including, binding with IP3 and Ca2+, clustering of IP3R, phosphorylation / dephosphorylation, and spatial distribution in the cytoplasm.
Activation of IP3R is begun by IP3 binding to the IP3 binding domain (amino acid 226-576) and Ca2+ ion permeating. At least four IP3 binding sites of the tetrameric structure of IP3R are essential to activate for IP3R (Taylor & Tovey, 2010). In addition, cytosolic Ca2+ was shown to regulate IP3, however, the effects of Ca2+ were biphasic. The modest increase of intracellular Ca2+ induces the enhancing response to IP3, while higher increase have inhibitory effects in IP3R1 through patch clamp electrophysiology of isolated Xenopus oocyte nuclei membrane (Mak et al., 2003). At least eight Ca2+-binding sites have been identified in IP3R1, Ca2+ promotes the transition from the square (or mushroom-like structure) to the windmill structure with relocation of four pheripheral IP3 binding domains (Hamada et al., 2003).
A commom consequence of activation of IP3R is the formation of IP3R clusters. IP3R clustering in somatic cells has been known to temporarily concur with increases in the cytosolic IP3 rathar than with [Ca2+]i (Lee et al., 2006b). Green fluorecence protein-tagged IP3R1 was expressed on the rat hippocampal neuron in vitro, and its lateral diffusion was regulated by actin filaments and 4.1N proteins, cytoskeletal-associated proteins enriched in neuron (Fukatsu et al., 2004). Single IP3R response first, then clustered IP3Rs open togerther with local Ca2+-puff, then all puffs lead to a global regenerative Ca2+ waves that spread out through whole cytoplasm in general somatic cells (Rahman, 2009, 2012). This IP3R clustering induced by IP3 production in the absence of a significant Ca2+ increase, but elevated Ca2+ without IP3 production did not induce IP3R clustering (Tateishi et al., 2005).
IP3R isoforms have multiple phosphorylation consensus sites and several docking sites for protein kinase and phosphatases on their sequences. Today at least 12 different protein kinases are investigated.
PKA phosphorylates two distinct sites on IP3R1 (S1588 and S1755) in the regulatory region, and phosphorylation of this sites regulates channel open probability of IP3R1 in whole cell patch clamp study (Wagner et al., 2008). Association of IP3R1 with protein phosphatase 1 alpha facilitates dephosphorylation of PKA mediated phosphorylation (Tang et al., 2003). Also, involvement of PKA to IP3R1 activation is mediated by leucine / isoleucine zipper domain in the regulatory region (Tu et al., 2004).
Another protein kinase G is shown resticted tissue distribution than PKA, could phosphorylate IP3R1 (Ser696) with IP3R-associated cGMP kinase substrate (IRAG) in microsomal smooth muscle membranes (Schlossmann et al., 2000).
Ca2+ / CaM-dependent protein kinase II (CaM KII) is a serine / threonin protein kinase expressed as an 8-12 monomers in most tissues, and highly sensitive to Ca2+ and calmodulin. Also it has been shown as Ca2+-oscillation decoder in varioius cellular response (Dupont & Goldbeter, 1998). All three different CaM KII inhibitors (KN-93, KN-62, and Autocamtide - 2 - Related Inhibitory Peptide (AIP)) inhibited ATP-mediated [Ca2+]i transient in calf pulmonary artery endothelialin vitro (Aromolaran & Blatter, 2005). Colocalizatioin of CaM KII and IP3R have reported in several tissues, this interaction induced phosphorylation of IP3R within N-terminal region. Besides, this phorylation of IP3R significantly decrease the open probability in lipid bilayers. It may suggest CaM KII acts on Ca2+ oscillations negatively (Zima et al., 2007).
The actilvity of conventional PKCs, including α, β, and γ, depend on Ca2+ and diacylgylcerol. Also, PKC activation lead to changes of subcellular localization of IP3Rs in many cell types (Vermassen et al., 2003). Phosphorylation sites for PKC on IP3R is [R/K]-X-[S/T]-X-[R/K], and counteracts by phosphatase calcineurin (Cameron et al., 1995). In addition, there are several protein to interact with PKC for IP3R activation, FKBP12, mTOR and RACK1.
All three IP3R isoforms contain an R-X-R-X-X-[S/T] consensus site for PKB in their C-terminal. PKB have a survival effects on apoptosis by inhibiting IP3-induced Ca2+ release on S2681 (Joseph & Hajnoczky, 2007).
Cyclin-dependent kinases (CDKs) are a family of proteinkinases and involved in cell cycle, including extracellular signal regulated kinase (ERK), and polo like kinase.
A well investigated cellular system, mammalian oocytes, was showed these protein kinases activity changes during oocyte maturation. Increase of [Ca2+]i during fertilization has been reported IP3R mediated Ca2+ release with single, large Ca2+ increase followed by Ca2+ oscillation for several hours until pronuclear formation. These Ca2+ changes depend on IP3R activity through protein kinase activity. CDKs (cdc2) assemble with the regulatory protein cyclin B to form the maturation promoting factor, which is major protein for starting of oocyte maturation (Polanski et al., 2012). CDK1 phosphorylates IP3R1 at S421, in IP3 binding domain and T799 in regulatory domain. Both sites are conserved in IP3R1 from human to Drosophila (Lee et al., 2006b). During oocyte maturation, IP3R1 undergoes cell cycle-dependent phosphorylation in mouse oocytes, M-phase kinases associates as regulators of IP3R1 in mammalian egg (Jellerette et al., 2004). CDK1/cyclin B mediated IP3R1 phosphorylation at T799 increases IP3 binding activity, resulting in an increase Ca2+-releasing activity.
ERK1 and ERK2 are cell cycle dependent kinases and phosphorylate an P-X-[S/T]-P. In mouse IP3R1 has three ERK1/2 phosphorylation sites for ERK1/2 at S436, T945 and S1765. From in vitro phosphorylation studies represent that ERK2 phosphorylates IP3R1 at S436, that mutation (S436A) abrogates IP3R1 phosphorylation (Lee et al., 2006a).
Phosphorylation of tyrosine residues of IP3R1 was observed in T-cell receptor activation, the IP3R phosphorylation is induced by tyrosine kinase, Fyn at Y353. This phosphorylation was reduced in thymocytes in fyn-/- mice (Jayaraman et al., 1996). In T-cell activation, IP3R phosphorylation by Fyn induces increase of affinity for IP3 to a 5 times, which means that IP3R1 can continue to release Ca2+ from intracellular Ca2+ store during the reducing of intracellular IP3 (Cui et al., 2004).
B-cell lymphoma 2 (Bcl-2), encoded in humans by the Bcl2 gene, is a proapoptotic and anti-apoptotic protein with four BH domain and embedded in the endoplasmic reticulum, nuclear envelope, and mitochondrial outer membrane (Greenberg et al., 2014). Bcl-2 proteins have been associated on PKA mediated phophorylation.
CHANGES OF IP3R1 DURING OOCYTE MATURATION IN MAMMALS
Multiple changes in Ca2+-response mechanism via IP3R1 including IP3R1 clustering on the plasma membrane (Mehlmann & Kline, 1994; Mehlmann et al., 1996; Machaca, 2004; Sun et al., 2011), phosphorlation of IP3R1 (Vanderheyden et al., 2009; Wakai et al., 2012; Zhang et al., 2015), have been described during oocyte maturation.
AsIP3R1 is observed in whole cytoplasm, 1.9 fold maturation associated increase was indicated by western blot analysis, in contrast, the type 2 and the type 3 IP3R were detect lesser in oocytes than IP3R1 (Fissore et al., 1999).
During the mouse oocyte maturation, it has known to increase an sensitivity to IP3 mediated release of intracellular calcium. 1.5 to 2 fold maturation associated increase in the mass of IP3R1 (Fissore et al., 1999) stands for this increase in IP3 sensitivity, and administrated dsRNA corresponding to the IP3R1 sequence into germinal vesicle (GV) immature oocytes and resulted in the mass of IP3R1 protein and significantly decreased Ca2+ transients in these oocytes than controls (Xu et al., 2003).
The sensitivity of Ca2+ release has known to increase during oocyte maturation from GV stage to MII stage (Mehlmann & Kline, 1994; Mehlmann et al., 1996). In immature GV oocytes showed homogeneous distribution of IP3R1 on entire cytoplasm except geminal vesigle. However, during oocyte maturation, IP3R1 reorganized their localization and clustering itself. Most of the IP3R1 distributed in whole cytoplasm with cortical clusters near plasma membrane in large clusters, 1-2 μm in diameter (yellow arrow in Fig. 3) (Mehlmann et al., 1996). Inhibition of this clustering by MEK-specific inhibitor, U0126, reduced phosphorylation of IP3R1 and calcium release during fertilization (Lee et al., 2006a; Ito et al., 2008). Changes in IP3R1 sensitivity may underline the changes of the spatio-temporal Ca2+ responses during oocyte maturation. The conductivity of IP3R1 to Ca2+ in response to increase in IP3 is enhanced at mature MII oocytes (Machaca, 2007; Ullah et al., 2007). The Ca2+ transient by PLCζ in GV showed very small and narrow spike, however, that in mature MII oocytes showed big and long (4-5 mins) spike in mouse oocytes (Machaca, 2007). Also Ca2+-oscillations in response to sperm of immature oocytes were maximum three much smaller transients, which ceased after 1 hr. But the oscillation of Ca2+ in mature oocytes by sperm continued for 4 to 5 hrs, near pronuclear (PN) formation (Jones et al., 1995a; Jones et al., 1995b). These sensitivity of Ca2+ response is controlled by M phase kinase during oocyte maturation with cell cycle dependent (Jones et al., 1995a; Jellerette et al., 2004). During oocyte maturation GV immature oocytes have nonphosphorylated form of IP3R1, on the other hands, MII mature oocytes have phosphorylated IP3R1 on S436 by MAPK, and S421, T799 (Lee et al., 2006b; Zhang et al., 2015).
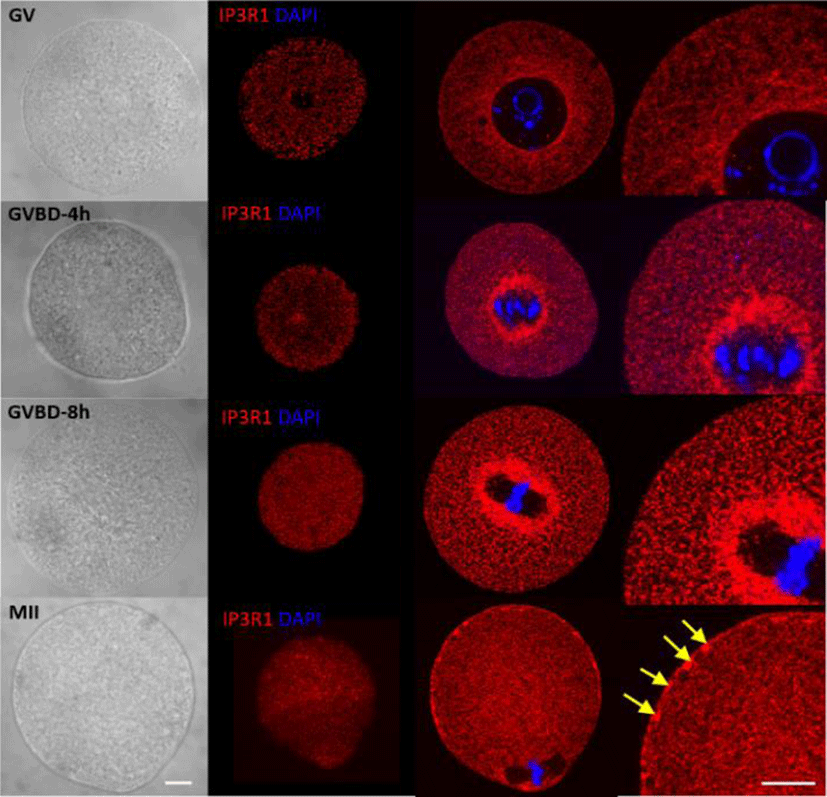
In mammalian oocytes, fertilization mediated Ca2+-oscillations are very active at the beginning of fertilization, which is ceased as zygotes come close to the pronuclear stage. Further, more than 50% of IP3R1 mass is downregulated by PN stage (Brind et al., 2000; Jellerette et al., 2000; Jones, 2005). These IP3R1 degradation requires continuous identical and persistantly elevated levels of IP3 by PLCζ (Jellerette et al., 2000). Also continuous degradation of the IP3R1 results in Ca2+-oscillation with reduced periodicity (Lee et al., 2010). Degradation of IP3R1 is mediated by ubiquitin-proteasome pathway after IP3 bindingin intact mammalian cells (Alzayady & Wojcikiewicz, 2005).
CONCLUSION
The generating of Ca2+-release during fertilization provides the widespread intracellular signaling for egg activation and further embryonic development. In mammalian oocytes, Ca2+ oscillation during fertilization persists for several hours until the pronuclear formation. Here we interpret general charateristic of IP3R1 and the role of IP3R1 during fertilization. Further studis should be investigated the molecular mechanism that controls the activation and degradation of IP3R1 in egg activation and further embryonic development. It is hoped that this work will helpful the understanding of physiological problem in infertility or subfertility patients.