INTRODUCTION
Adipogenesis from precursor cells has been extensively studied in order to overcome the physiological problems of obesity and its related diseases. This differentiation and homeostasis is under the control of various factors and complex processes (Cha et al., 2013; Park et al., 2013a,b). Recently vigorous studies revealed various adipogenic factors such as dexamethasone, 3-isobutyl-1-methylxanthine, Dexras1, glucocorticoid, Notch 4, alpha-2-macrogloobulin, BMP4, Pref-1, paraoxonase/arylesterase 1, retinol, preadipocyte factor-1, Wnt (Cha et al., 2013; Lai et al., 2013; Muenzner et al., 2013; Park et al., 2013a; Gustafson & Smith, 2012; Seres et al., 2010; Ross et al., 2000; Yeh et al., 1995; Smas & Sul, 1993).
Adipocytes play a central role in maintaining lipid homeostasis and energy balance in vertebrates by storing triacylglycerols (TAGs) or releasing free fatty acids in response to changes in energy demands (Fruhbeck et al., 2001). However, the excessively grown adipose tissue in obesity is a major risk factor for a number of disorders, including diabetes, hypertension, and heart disease (Lee et al., 2005). In addition, the economic costs associated with being overweight and obese are rising continuously and pose social problems (Fu et al., 2005b; Lee et al., 2005; Farmer & Auwerx, 2004). The development of obesity is characterized by increased numbers of fat cells and their lipids due to the processes of mitogenesis and differentiation of adipocyte precursors, which are regulated by genetic, endocrine, metabolic, neurological, pharmacological, environmental, and nutritional factors (Fu et al., 2005a,b; Farmer & Auwerx., 2004; Wang et al., 2001). Accordingly, an understanding of the mechanism through which a particular nutrient affects the mitogenesis of preadipocytes and their differentiation to adipocytes would help prevent the initiation and progression of obesity and its associated diseases in humans (Wang et al., 2001).
Brown algae synthesize and accumulate variety of biomolecules such as xanthophyll, pigments, fucoidans, phycocolloids, and phlorotannins. Several studies concerning these types of compounds have pointed out the variety of biological benefits, including antioxidant, anticoagulant, antihypertension, antibacterial, and antitumor activities. Ishige okamurae (Phylum Phaeophyta, Class Phaeophyceae, Order Chordariales, Family Ishigeaceae), an edible brown algae forming persistant populations in clear water, has been collected from the coast of Jeju island, Korea (Lee & Kang, 1986). The extract of I. okamurae has been shown to have a variety of biological effects, including antioxidant effects (Kim et al., 2009), anti-viral effects (Ahn et al., 2002), apoptosis induction effect (Park et al., 2013b), and hypoglycemic effects (Min et al., 2011).
One of the known functions of the compound of I. okamurae is antiobesity (Jeon et al., 2013; Park et al., 2013a). Such antiobesity function is also known in the key compounds of some edible brown algae or extracts of them, such as Laminaria japonica (Shirosaki & Koyama, 2011), Petalonia binghamiae (Kang et al., 2012), Undaria pinnatifida (Kim & Lee, 2012). However, the role of the compounds of I. okamurae extract in adipogenesis is not much uncovered. In the present study, we examined the effects of the extract of I. okamurae on the differentiation of preadipocyte to adipocyte and accumulation of lipids.
MATERIAL AND METHOD
The extract of Ishige okamurae was performed at Seojjin Coorporation according to the established method (Heo et al., 2009). Briefly, the dried I. okamurae was exacted three times with 80% methanol and filtered. Then the filtrate was under evaporation at 40°C. I. okamurae methanol extract was suspended on distiled water and the partitioned with ethyl acetate. The ethyl acetate fraction was subjected to silica gel and Sephadex-LH 20 column chromatography.
3T3-L1 fibroblast cells from the American Type Culture Collection (ATCC, USA) were maintained at 37°C in an incubator with a humidified atmosphere of 5% CO2 and 95% air. 3T3-L1 was cultured in Dulbecco’s modified Eagle’s medium-high glucose (DMEM-HG) (Gibco Cat# 12800-58) containing 10% bovine calf serum (FCS) (Gibco Cat# 16170), 0.1 mg/ml streptomycin, 100 U/ml penicillin and 3.7 mg/ml sodium bicarbonate. Cells were seeded with 2.5×103 cells/cm2 density in 100Φ cell culture dish. To prevent cell differentiation, cells were maintained at subconfluent levels. Medium was changed every 2-3 days.
The effect of the extract of Ishige okamurae on the viability of cells was determined using the [3-(4,5-dimethylthiazol-2-yl)-2,5-diphenyltetrazolium] bromide (MTT) assay kit from Trevigen Inc. (Cat# 4890-025-01), which is based on the reduction of a tetrazolium salt by mitochondrial dehydrogenase in viable cells (Carmichael et al., 1987). Cells were subcultured at 96-well plate, after 6 hr, cells were treated with caffeine (0.05 mM and 0.1 mM) and the extract of I. okamurae (0.4 μg/ml, 2 μg/ml, 10 μg/ml, 50 μg/ml). Then, 44 hr later, added 10 μl of the MTT reagent and 2-4 hr later (until purple dye is visible.) added 100 μl of the Detergent Reagent to all wells. After incubation for 2 hr, the optical density measured on 570 nm with spectrophotometer. Caffeine was used as a positive control in this study.
Culture and differentiation induction of 3T3-L1 cells was performed as described in ATCC (Student et al., 1980). Briefly, cells were subcultured with 2.5×103 cells/cm2 density in 24-well plate in DMEM-HG containing 10% fetal calf serum (FCS). Induction was started after 2 days of 100% confluence. Media was changed with differentiation day 0, differentiation medium (DMEM supplemented with 10% fetal bovine serum (FBS), 0.5 mM methylisobutylxanthine (IBMX), 1.7 μM insulin, and 1.0 μM dexamethasone). Caffeine (0.05 mM and 0.1 mM) and the extract of I. okamurae (0.4 μg/ml, 2 μg/ml, 10 μg/ml, and 50 μg/ml) were added in the differentiation media to reveal their roles in adipogenesis. After 2 days, media were changed with adipocyte maintenance medium (DMEM supplemented with 10% FBS, 1.0 μg/ml of insulin). Then, medium was replaced every 2-3 days until 10 days. We measured the degree of differentiation by Oil Red O staining and optical density.
3T3-L1 cells were maintained in noninductive control medium until 90-95% confluent the culture plate. The expression profiles of the adipogenesis marker genes were analyzed. Total RNAs from the cells on day 5 and ells were isolated using TRIzol Reagent according to the manufacturer’s instructions. The purity of RNA was assessed by determining the ratio of absorbance at 260 nm to that at 280 nm. First strand cDNA was synthesized using First-Strand synthesis kit (Stratagene, Cat #. 200420) according to the manufacturer’s instructions. Briefly, the mixtures were incubated at 65°C for minutes and place tube at room temperature for 10 minutes for the primers to anneal to the RNA. And incubated at 42°C for 60 minutes and incubated at 70°C for 15 minutes to terminate cDNA synthesis. Quantitative real-time PCR was performed for peroxisome proliferator activated receptor gamma (Pparg), lipoprotein lipase (Lpl), adiponectin (Adipoq), and acidic ribosomal phosphoprotein P0 (36B4, Rplp0). Rplp0 was used as internal control. The primers (Bioneer, Koear) were depicted in Table 1.
After induction, the cells were washed with phosphate-buffered saline (PBS). The cells were fixed with 0.4% formaldehyde in room temperature for 10 min, and then briefly washed with H2O. The cells were stained with freshly prepared Oil Red O solution for 1 hr. The morphology was analyzed with microscope. To measure the stained lipids, Oil Red O dye were eluted with isopropanol. After elution, the solution was transferred to 96-culture dish and measured the optical density (500 nm) with microplate reader.
RESULT
The effects of the extract of I. okamurae on the viability of 3T3-L1, a preadipocyte, were analyzed with MTT assay as mentioned in Materials and Methods. As expected, the viability of 3T3-L1 was not inhibited by caffeine. The viability was significantly higher (p<0.05) in 0.1 mM caffeine treated cells (Fig. 1). However, in all groups treated with extract of I. okamurae (0.4-50 μg/ml), the viability of 3T3-L1 was significantly higher (p<0.05) than the vehicle. In the case of 10 μg/ml group, the viability was significantly higher than that of 0.1 mM caffeine group (Fig. 1).
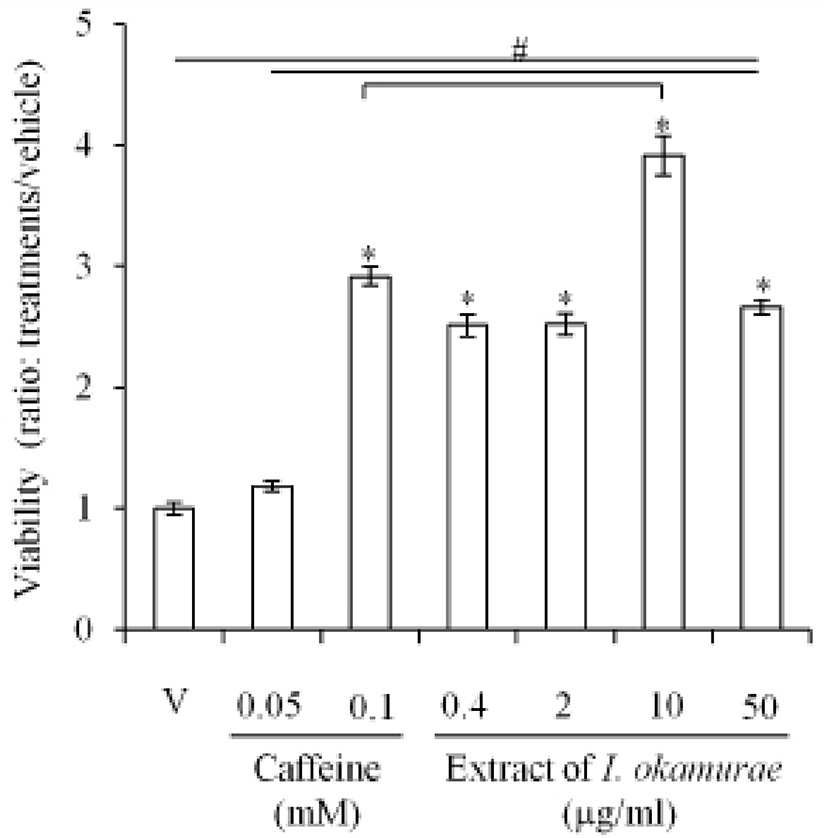
Lipids accumulated in the cells which were induced for adipogenesis from 3T3-L1 preadipocyte (Fig. 2A). Caffeine was used as a control for the inhibition of adipogenesis of 3T3-L1 and accumulation of lipids in differentiating cell. As expected, Oil Red O was stained in most cells of vehicle (Fig. 2A2). The specific ranges of concentration (0.4-2 μg/ml) of I. okamurae extract did not inhibit accumulation of lipids in the differentiated cells (Fig. 2A2,3). In 10 μg/ml I. okamurae extract group, the number of cells which accumulate lipids was little bit decreased but not distinguishable (Fig. 2A4). However, the number of cells which was accumulated with lipids was decreased in 50 μg/ml I. okamurae extract (Fig. 2A5).
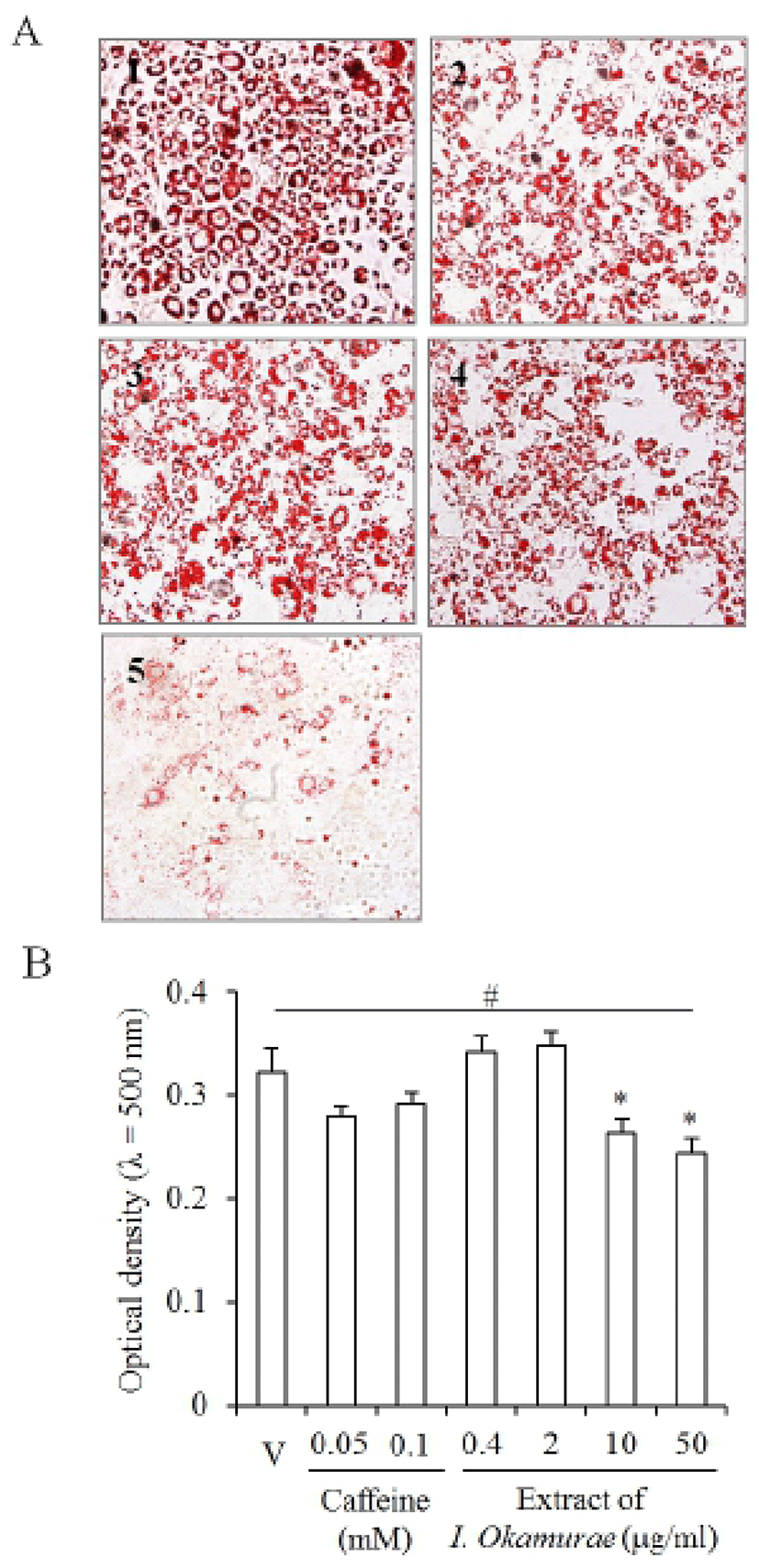
Interestingly, the optical density of the extracted Oil Red O from the stained cells was significantly decreased both in 10 and 50 μg/ml I. okamurae extract. However, the optical density was increased in the low concentration groups (Fig. 2B). In the caffeine control, the optical density was not affected: there was no statistical significance (Fig. 2B). These results suggested that the differentiation or accumulation of lipids is modified by the extract of I. okamurae.
Based on the morphology of the differentiated cells, we analyze the expression profiles of some of the well known adipogenic marker genes. Its expression was increased by the adipogenesis induction of 3T3-L1 preadipocyte. The expression profiles of Pparg mRNA in the group treaed with I. okamurae were different from the vehicle and caffeine treated groups (Fig. 3A). The expression levels of Pparg mRNA were not different from the vehicle both in 5 days and 10 days of induction. The levels of Pparg mRNA expression were decreased by the extract of I. okamurae in a concentration dependent manner until 5 days of induction. The expression levels continuously maintained until 10 days of induction in control and caffeine treated cells, but their levels were decreased after 5 days of induction in the I. okamurae extract treated cells. Especially, the expression levels were significantly decreased in 50 μg/ml I. okamurae extract both 5 and 10 days of induction (Fig. 3A).
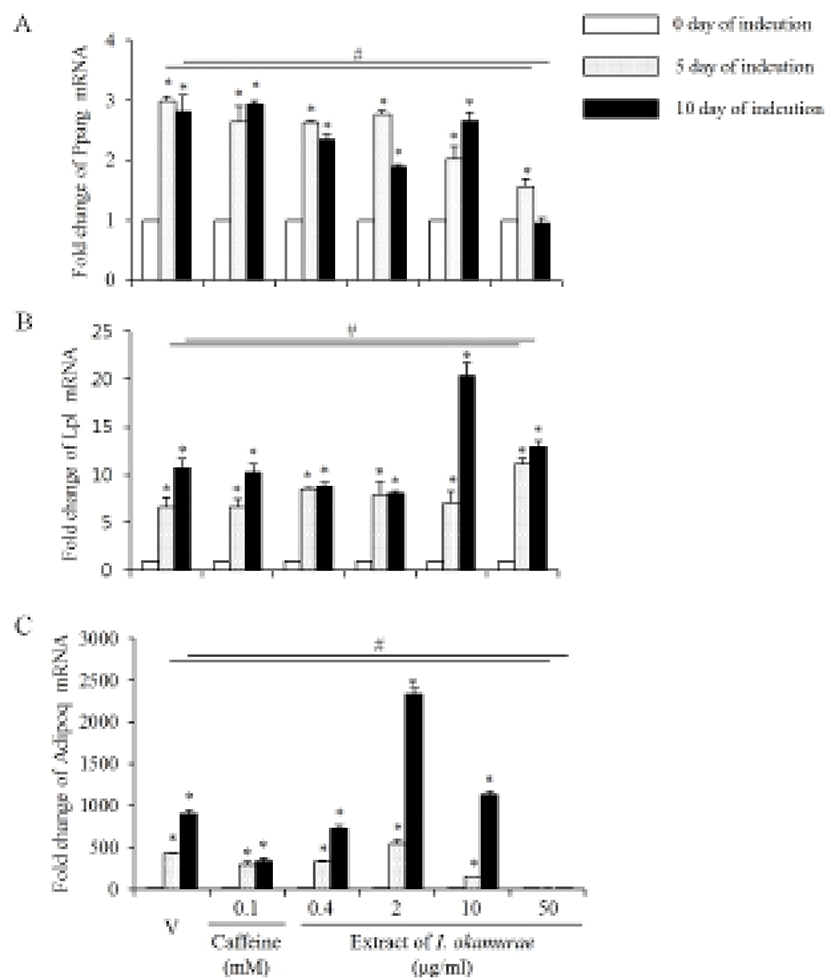
Lp1 mRNA expression was modified by the extract of I. okamurae. Its expression was increased by the adipogenesis induction of 3T3-L1 preadipocyte. On day 5 of adipogenesis induction, the expression levels of Lpl mRNA were similar between the caffeine and 0.4-10 μg/ml I. okamurae extract, and the vehicle treated cells. However, its expression level was significantly high in 50 μg/ml I. okamurae extract treated cells on day 5 of induction. The expression of Lpl was continuously increased in all groups until day 10 of adipogenesis induction. Lp1 mRNA expression levels were significantly higher in 50 μg/ml I. okamurae extract both 5 and 10 days of induction (Fig. 3B).
The expression profiles of Adipoq mRNA were also modified by the extract of I. okamurae. Its expression was increased by adipogenesis induction of 3T3-L1 preadipocyte. On day 5 of adipogenesis induction, the expression levels were significantly low in all groups except 2 μg/ml I. okamurae extract treated group. Its expression levels continuously increased only in all caffeine treated groups and 0.2-0.4 μg/ml I. okamurae extract treated group. On day 10 of adipogenesis induction, the expression levels were significantly increased in 2 and 10 μg/ml I. okamurae extract treated group. In 50 μg/ml I. okamurae extract treated cells, the expression levels of Adipoq were lower than that of control and other groups both in 5 day and 10 day of adipogenesis induction (Fig. 3C).
DISCUSSION
Obesity became a social problem in industrialized countries and many studies extremely tried to solve that problem. However, it is until now far from the goal. The physiological problems derived from overaccumulation of nutrients are one of the major disorders. During development, increase of the number of adipocyte and the size of adipocytes in a specific body site is a way to forming organ and body shapes. Increases in the size of adipose tissue is achieved both by increase in the number of adipocyte and the differentiation of preadipocyte to adipocyte. Growth of white adipose tissue and defects in adipogenesis are critical in energy balance and production of signal molecules (Xue et al., 2013). Hypertrophic obesity in the abdominal subcutaneous tissue is characterized by a reduced recruitment of new adipose cells by the reduced preadipocytes (Amer et al., 2010; Spalding et al., 2008). It is suggested that reduction in the recruited preadipocyte is due to an apparent genetic impairment in the ability to recruit and differentiate (Gustafson & Smith, 2012; Arner et al., 2011; Jansson et al., 2003). Therefore, it is important to keep the optimal number of preadipocyte or adipose stem cell for health life. It has been tried to regulate the growth of adipose tissue through several ways such as suppression of food intake and digested energy source absorption, inhibition of preadipocyte proliferation and of differentiation. So far, not much is known for the mechanisms for commitment of mesenchymal stem cells to preadipocytes, but the differentiation of preadipocytes to adipocytes is cleared. Differentiation of preadipcyte is regulated by complicated networks of transcription factors, including CCAAT/enhancer-binding proteins and peroxisome proliferator-activated receptor γ protien (PPARγ) (Chen et al., 2013a; Lefterova & Lazar, 2009; Tontonoz & Spiegelman, 2008).
The suppression of the activity of antioxidant response element (ARE) with isoniazid interrupts the transcriptional network of adipogenesis and leading to impaired adipogenic differentiation (Chen et al., 2013b). Recently, it is suggested that utilization of natural sources is helpful to reducing obesity. We performed this study to get possible approach reducing adipose tissue size or growth with natural sources. One of the well established model in the study of differentiation and triglyceride accumulation is 3T3-L1 preadipocyte (Chen et al., 2013a,b). The cellular activity was significantly increased in the cells treated with the extract of I. okamurae compared with the control. Caffeine also increased the cellular activity in concentration dependent manners. High level of ATP in preadipocyte means that cells can achieve proliferation or differentiation.
Low concentration of I. okamurae extract did not inhibit accumulation of lipids in the differentiating cells but high (50 μg/ml) I. okamurae extract inhibited lipid accumulation. It means that the differentiation or accumulation of lipids is modified by the extract of I. okamurae in a concentration dependent manner. Differentiation of preadipocyte to adipocyte requires the concerted regulation of gene expression by various adipogenic factors. Among them, hormone nuclear receptor PPARγ plays central roles by controlling down stream adipogenic genes (Farmer, 2006; Tontonoz et al., 1994). The expression levels of Pparg mRNA were decreased by the extract of I. okamurae in a concentration dependent manner until 5 days of adipogenesis induction. Especially, the expression levels were significantly decreased in 50 μg/ml I. okamurae extract until differentiation. Adipoq encodes adiponectin which is involved in the regulation of glucose level and breakdown of fatty acid. The roles of adiponectin also include the promotion of adipocyte differentiation and lipid accumulation (Fu et al., 2005a). The expression levels of Adipoq mRNA were significantly decreased during adipogenesis by high concentration of the extract of I. okamurae. Lpl encodes liporotein lipase which has dual functions of triglyceride hydrolase and ligand/bridging factor for receptor-mediated lipoprotein uptake. Lpl expression is increased during adipogenesis (Ullah et al., 2013). The expression levels of Lp1 mRNA were increased until day 10 of adipogenesis induction. Based on these results we can suggest that the extract of I. okamurae can suppress preadipocyte differentiation and accumulation of lipids in a concentration dependent manner without reducing viability of preadipocytes. In murine 3T3-L1 preadipotyes, caffeine does not inhibit differentiation to adipocyte but it suppresses the intracellular lipoid accumulation (Riedel et al., 2012; Nakabayashi et al., 2008). In the result, caffeine did not inhibit Pparg and Lpl mRNA expression, but Adipoq mRNA expression. Base on them, it is suggested that the extract of I. okamurae contain compound decreasing the competence of preadipocyte for adipogenesis.
With 3T3-L1 model, it is revealed that Wnt/b-catenin signaling pathway is a key signaling pathway in differentiation of 3T3-L1 cells into adipocytes (Chen et al., 2013a). Notch4 signaling pathway is known adipogenesis pathway. It promotes facilitated differentiation of 3T3-L1 cells through modulating the ERK pathway and up-regulating adipogenic genes such as C/EBPα, PPARγ, aP2, LPL, and HSL during the middle and late stages of 3T3-L1 adipogenesis (Lai et al., 2013). So far, it is not explored what is the mediator of the work of the extract of I. okamurae or its key molecules. However, it is clearly revealed that the extract of I. okamurae have antiobesity role without reducing the viability of preadipocyte, 3T3-L1. It has various important meaning for such as treatment obesity with conserving preadipocte. In addition, it will provide a plausible way to regulate the growth of adipose tissue. Further investigations will clarify the current situation which is ongoing in our group.