INTRODUCTION
Embryonic stem (ES) cells established from the inner cell mass (ICM) of pre-implanted blastocysts are pluripotent cells with the ability to long-term proliferation in a state of undifferentiation (Park et al., 2004). And, these cells can differentiate to all types of body cells and lineages derived from three embryonic germ layers, named the endoderm (Schroeder et al., 2006; Kim et al., 2007), mesoderm (Sachinidis et al., 2003) and ectoderm (Lee et al., 2000; Han et al., 2008) in vitro or in vivo. Stem cell studies provide knowledge about early embryogenesis (Robertson, 1991), gene targeting (Zheng et al., 1999), and regenerative medicine (Dinsmore et al., 1996).
To culture stem cell in the undifferentiated state for long time, there are many previous studies. For example, adding regulator such as activator or inhibitor in pluripotency related signaling pathway (Hila et al., 2012; Ariel & Ali, 2006) and regulate culture environment like culture on feeder cell layer, use of feeder cell conditioned medium and hypoxia during short time (Gail, 1981; Yue et al., 2015; Warren et al., 2007). Feeder cell layer has been used in general on stem cell culture. There are many kinds of cell lines used as feeder cell layer. Fibroblasts like mouse embryonic fibroblast (MEF) cell and SIM mouse embryoderived thioguanine and ouabain resistant (STO) cell lines were most used to establish and culture of ES cell (Thomas et al., 1985; Gail, 1981). Cells derived umbilical cord blood cell or adult bone marrow cell, also, were used as feeder cell layer (Ye et al., 1994; Linzhao et al., 2003).
In this study, we compared the effects of two kind feeder cells, MEF cell and STO cell, on maintenance of stemness in mouse ES (mES) cell. The ES-D3 (D3) cell line, one of the well-known mES cell line, is used. We checked colony formation, AP activity, identification of pluripotency markers of D3 cell colonies. Our results provide helpful information for maintaining characteristics of stem cell.
MATERIALS AND METHODS
MEF cell was prepared from fetus in uteri of 13.5-day pregnant CDF1 mice and STO cell line was purchased from American type culture collection institute (ATCC; Manassas, VA, U.S.A.). MEF and STO cells proliferated on 100-mm culture dishes coated with 0.1% gelatin in a 5% CO2 incubator at 37.0°C with cell culture media (CM) consist of Dulbecco’s modified eagle medium (DMEM; Gibco, Grand Island, NY, USA) supplemented with 10% Fetal bovine serum (FBS; Hyclone, Logan, UT, USA), 100 mM non-essential amino acids (NEAA; Gibco), 0.55 mM β-mercaptoethanol (Gibco), 1% Antibiotic-Antimycotic (Gibco). Proliferated MEF and STO cells were mitotically inactivated by treatment of 10 μg/mL mitomycin-C (MMC; Sigma) in CM for 2~3 hours. Then, the cells were washed with PBS 3 times, treated 0.5% trypsin-EDTA for 3 min, centrifuged at 2,000 rpm for 2 min and reseeded on 0.1% gelatin coated 60-mm dishes in stem cell culture medium (SM) containing same reagent and concentration except 20% FBS. Feeder cell layers were used to culture of D3 cells after over-night (O/N) stabilization.
After stabilization of feeder cell layers, D3 cells purchased from ATCC and cryopreserved in LN2 were thawed on MEF feeder cell layer (D3/MEF), STO feeder cell layer (D3/STO) or 0.1% gelatin coated dish (feeder free, D3/-) and cultured in a 5% CO2 incubator at 37.0°C with SM containing 1,000 IU/mL leukemia inhibitory factor (LIF; Millipore, Bedford, MA, USA). D3 cell colonies formed from each groups were picked up and sub-cultured or analyzed.
After 4 days of culture, the AP activity, one of the stem cell marker most widely used, was examined in each group. The AP assay was performed using the Sigma 86C-1 kit according to the manual provided with the kit. Briefly, D3 cell colonies were washed twice with PBS, fixed in 80% (v/v) ethyl alcohol for 15 min at 4°C, washed again twice with PBS, and then stained with Fast blue BB salt/naphthol AS-BI for 15 min (Sigma) at room temperature (RT).
mRNA was isolated from D3 cell colonies using the Dynabeads mRNA Direct Kit (DynalAsa, Oslo, Norway). First-strand cDNA synthesis was achieved by the reverse transcription of mRNA using an oligo(dT)20 primer and SuperScript II reverse transcriptase (Invitrogen, Grand Island, NY, USA). cDNA samples were subjected to PCR amplification with following primers: mouse Gapdh sense 5’-CCCATGTTTGTGATGGGTGT-3’ and antisense 5’- CCTTCCACAATGCCAAAGTT-3’ (NM_008084, 180 bp); mouse Pou5f1 sense 5’-CTAGAGAAGGATGTGGTTCG 3’ and antisense 5’-TCAGGAAAAGGGACTGAGTA-3’ (NM_013633, 214 bp); mouse Nanog sense 5’-TGAGA TGCTCTGCACAGAGG-3’ and antisense 5’-CAGATGC GTTCACCAGATAG-3’ (NM_028016, 469 bp); mouse Sox2 sense 5’-GGAGTGGAAACTTTTGTCC-3’ and antisense 5’-GGGAAGCGTGTACTTATCCT-3’ (NM_011443, 154 bp). The PCR conditions were as follows: 34 cycles of denaturation at 94°C for 30 sec and annealing at 54°C for 30 sec extension at 72°C for 30 sec and a final extension of 5 min at 72°C. Samples were then cooled to 4°C. The PCR products were size-fractionated by 1.2% agarose gel electrophoresis and visualized by Redsafe staining. The final analysis was conducted in an image analyzer.
The immunocytochemistry protocol was basically the same as the one described previously (Kim et al., 2012). Briefly, after 3 days of culture, D3 cell colonies were fixed in 4% para-formaldehyde (PFA) at 4°C for O/N and then permeabilized with 0.1% Triton X-100 for 1 hr at RT. To prevent aspecific binding of antibodies (Abs), the cells were treated with 10% normal goat serum (VECTOR, USA) for 1 hr at RT and then treated with primary Abs during O/N at 4.0°C. The primary Abs were used anti-POU5f1 (Millipore, 1:10), anti-NANOG (Santa Cruz, Dallas, Texas, USA 1:10), anti-SOX-2 (Santa Cruz, 1:10) and anti-stage specific embryonic antigen 1 (SSEA-1, Santa Cruz, 1:50). The used secondary Abs were Alexa Fluor 546 conjugated goat anti-mouse immunoglobulin G (IgG) (SSEA-1, POU5f1), Alexa Fluor 546 conjugated goat anti-rabbit IgG (for NANOG, SOX-2) at a dilution of 1:100 by PBS. Nuclei were stained with 5 μg/mL of 4’-6-diamidino-2-phenylindole (DAPI). Cells were observed with an inverted Olympus IX-71 (Japan) microscope equipped for epifluorescence.
The general linear model (GLM) procedure within the Statistical Analysis System (SAS User’s Guide, 1985, Statistical Analysis System Inc., Cary, NC. USA) was used to analyze data from all experiments. A paired Student’s t-test was used to compare relative gene expression. P values of <0.05 were considered significant.
RESULTS
Before the ES cell culture, differences of morphology of MEF and STO feeder cells were checked (Fig. 1). MEF cells grew up looser than STO cells (Fig. 1A, C). More detail (Fig. 1B, D), MEF cells presented irregular shapes and the cytoplasm of MEF cells is wide. While, STO cells presented rhombus like regular shapes, and the cell size was smaller than MEF cells.
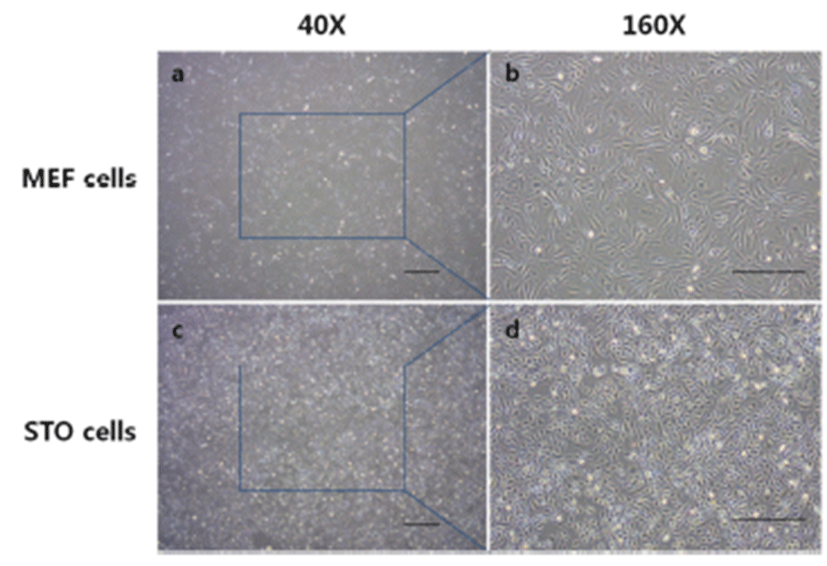
To compare the colony formation of D3 cell on different feeder layer, D3 cells were culture on MEF feeder layer or STO feeder layer and feeder free. There were no differrences (Fig. 2). Most D3 cells were assembled to round dorm-shape colonies, and the cell to cell boundaries were not clear.
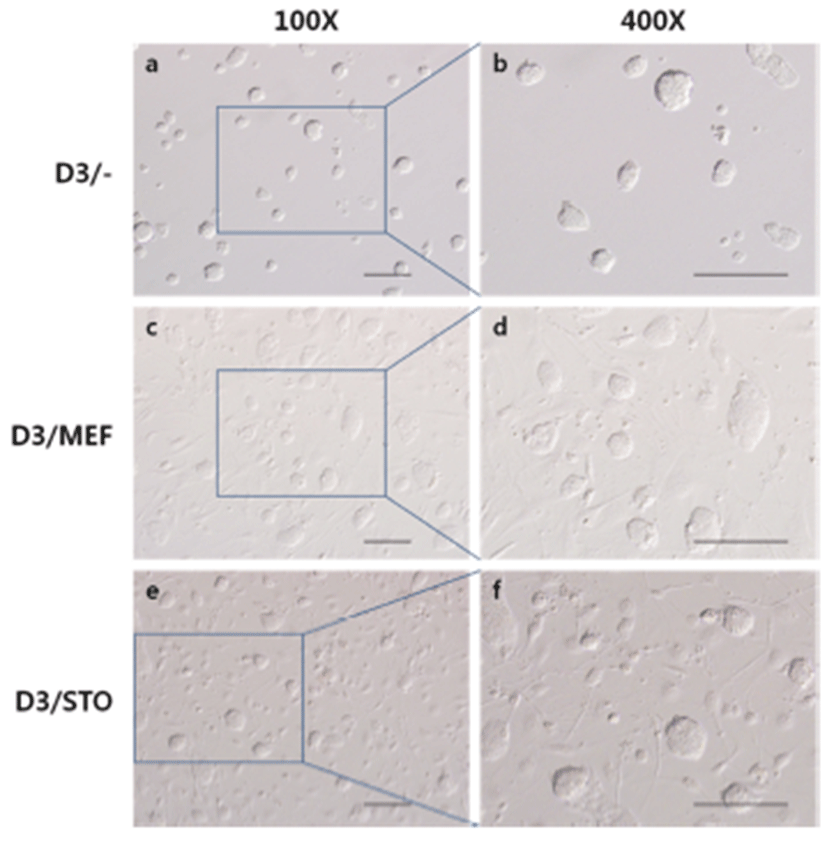
To check the phenotypic pluripotency, we performed AP assay with D3 cell colonies on MEF or STO feeder cell layer. AP activities presented violet color under a visible ray were highly detected in D3/MEF, D3/STO and D3/– and there was no differences among groups.Fig . 3
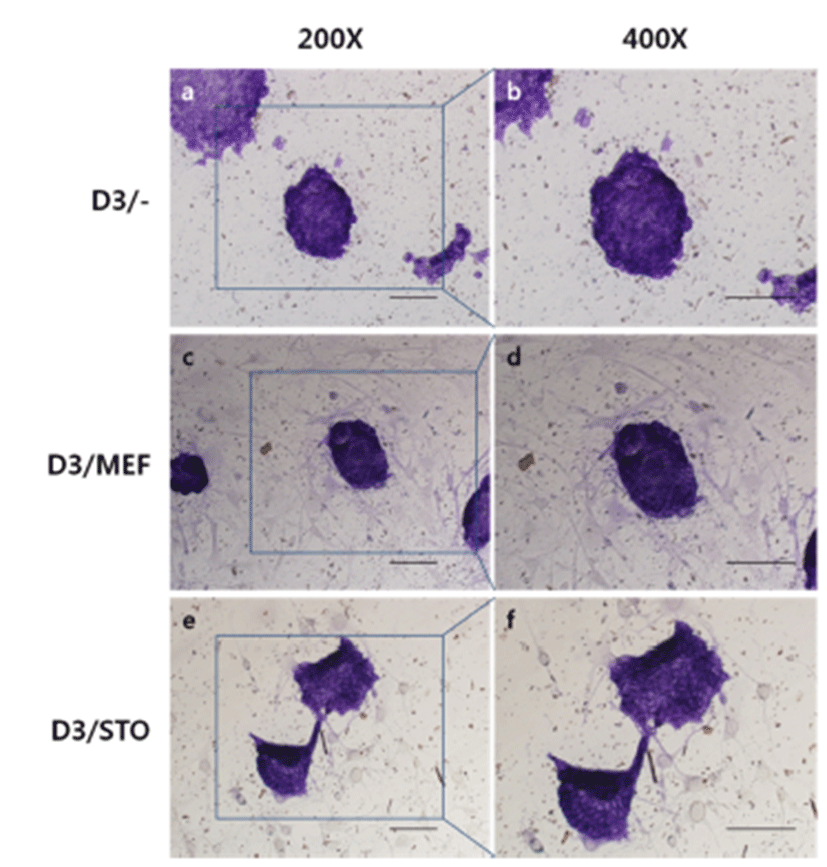
The mRNA expression of pluripotency-related genes (Pou5f1, Nanog and Sox2) was analyzed by RT-PCR (Fig. 4). The mRNA transcript of Pou5f1, Nanog and Sox2 was expressed among D3/–, D3/MEF and D3/STO groups. Although the Pou5f1 transcript was similar gene expression among the three groups (D3/–, 65; D3/MEF, 79 and D3/STO, 61, Fig. 4A and B), the Nanog and Sox2 transcripts were the highest gene expression in D3/MEF (79 and 93) compared to both D3/STO (61 and 77) and D3/– (65 and 81). However, there was no difference between D3/STO and D3/–.
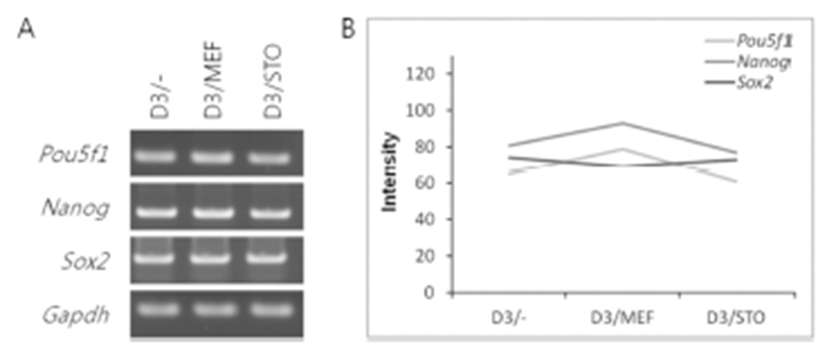
To confirm the synthesis of pluripotency marker protein, POU5f1, NANOG, SOX-2 and SSEA-1 was performed immunocytochemistry. Localization of POU5f1, NANOG, SOX-2 and SSEA-1 was positively detected in all groups, D3/MEF, D3/STO and D3/– (Fig. 5). Although the activity of POU5f1 in D3/– was similar to D3/MEF and D3/STO, the activity of POU5f1 in D3/MEF was higher than D3/ STO (D3/–, 82.0±15.81; D3/MEF, 78.4±6.39 and D3/STO, 58.4±7.30, Fig. 5A and B, P<0.05). The activity of NANOG in D3/MEF was increased compared to both D3/STO and D3/– (D3/–, 55.0±4.64; D3/MEF, 72.8±7.69 and D3/STO, 32.0±4.30, Fig. 5A and B, P<0.05). The activity of NANOG in D3/STO was lower than D3/– (P<0.05). The activity of SOX-2 in D3/MEF was increased compared to both D3/ STO and D3/– (D3/–, 62.0±6.20; D3/MEF, 81.2±3.56 and D3/STO, 56.0±4.90, Fig. 5A and B, P<0.05). However, there was no difference between D3/STO and D3/–. The activity of SSEA-1 was no difference in three groups (D3/–, 57.4±15.07; D3/MEF, 59.0±9.43 and D3/STO, 55.4±12.18, Fig. 5A and B, P<0.05).
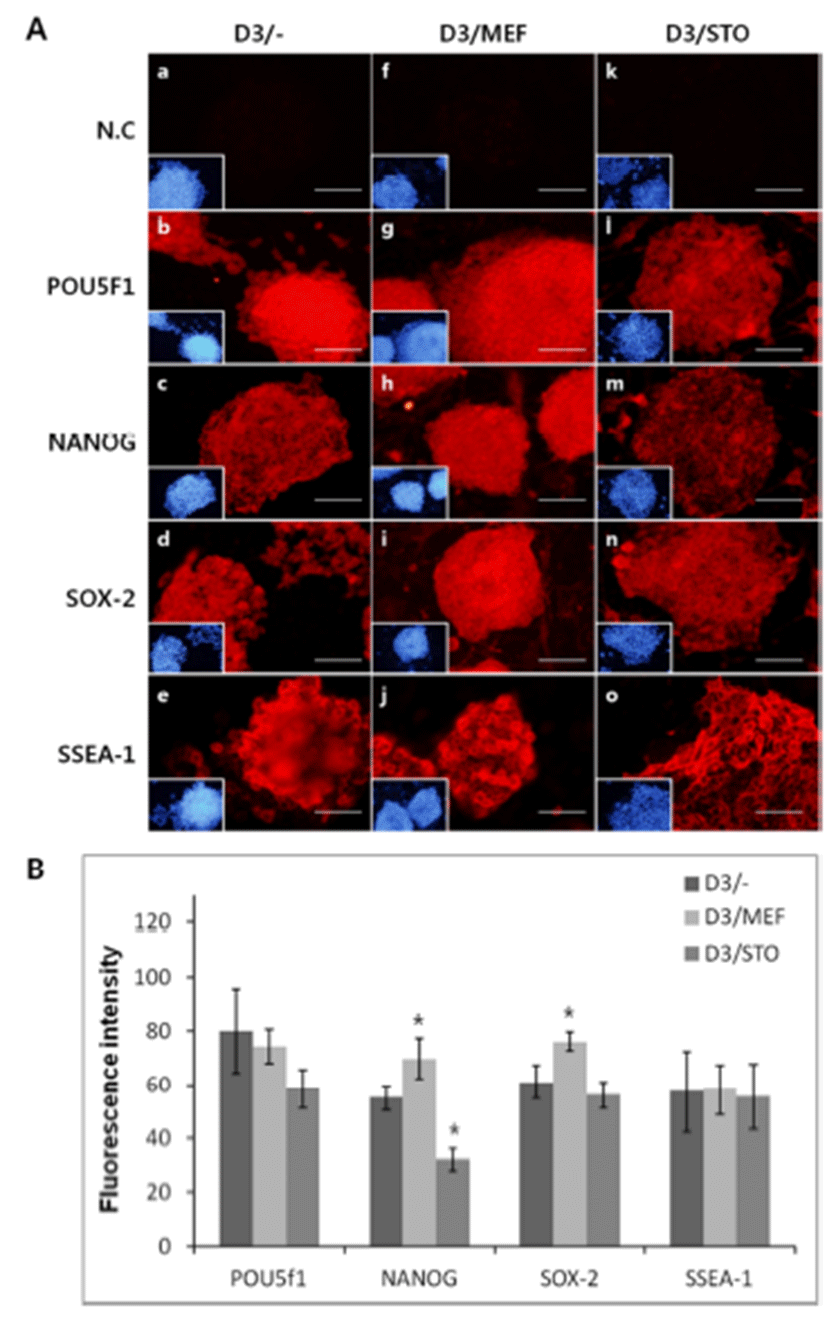
DISCUSSION
To efficient culture of embryonic stem cells, the proper feeder cell layer is important. In present study, we investigated differences of effect by feeder cell types to mouse embryonic stem cell culture. We undertook to characterize and compare the ability of D3 cell colonies cultured on MEF, STO cell feeder layer or feeder free. This characterization was performed based on colony formation, AP activity and expression of pluripotency marker. There were no differrence on colony morphologies and AP activities in D3/MEF, D3/STO and D3/–. However, the D3/MEF has not only increased pluripotency marker genes, Nanog, Sox2 expression, compared to D3/STO and D3/– but also their protein synthesis of D3/MEF were highly detected than D3/STO or D3/–.
Culture of stem cells on feeder cell layer is regarded good strategy because feeder cell layer helps stem cell. Feeder cells secrete extracellular matrix containing some factors into culture medium. The factors help attachment and survival, promote self-renewal and suppress differentiation of stem cells (Kenichiro et al., 2006). MEF cell is a kind of fibroblast derived from mouse fetus. It extensively used stem cell culture (Denning et al., 2006; Gómez et al., 2010). But, it is necessary for ES cell culture to isolate MEF cells from mouse fetuses repeatedly because MEF cells have limited proliferation potential (Park et al., 2003). As an alternative, STO cell line is used for feeder cell (Park et al., 2003; Park et al., 2004). STO cell line was immortalized SIM-mouse embryonic fibroblasts. Therefore, they are useful feeder cells for mES cell culture.
The morphologies of stem cell colonies are easiest distinguishable marker of stemness. Good quality ES cell colonies are present dorm-shape morphologies having unclear cell to cell boundaries by compactly clustering and repeatedly proliferating (Kim et al,. 2012; Park et al., 2004). In our study, the D3 cell colonies presented the morphologies described above. There were no differences among the groups. It indicates the stemness of D3 cells are maintain in all groups. AP expression is a suitable marker of pluripotent stem cells. The low level of AP activity denotes the restriction of pluripotency (Katerina et al., 2015). Our results indicated that D3/MEF, D3/STO and D3/– have high level of AP activity. In previous study, AP staining has been used to indicator of undifferentiation in human iPS cell (Julie et al., 2011). We suggest that feeder cell type could not affect undifferentiated state of embryonic stem cell.
Interestingly, our data shows that the mRNA expression of pluripotent genes such as Nanog and Sox2 increased on D3/MEF compared to D3/STO or D3/– while Pou5f1 expression is similar. Although SSEA-1 has no difference on among D3/MEF, D3/STO and D3/– in protein level, POU5f1, NANOG and SOX-2 significantly increased on D3/MEF. The function of POU5f1 and SOX-2 as core transcription factors to determine pluripotency is well documented (Boyer et al., 2005; Wang et al., 2006). They synergistically upregulate stemness genes, while suppress differentiation-associated genes in both mouse and human ES cells (Takahashi et al., 2007). NANOG sustains selfrenewal and the undifferentiated state is through the modulation of POU5f1 and SOX-2 levels. These two transcription factors in turn control the downstream genes important for maintaining pluripotency or inhibiting differrentiation (Loh et al., 2006). The antigen SSEA-1, also known as CD15 or LeX, is a fucose-containing trisaccharide highly expressed on ES cells in the developing brain and adult subventricular zone (Capela & Temple, 2002; 2006). SSEA-1 positive cells exist throughout the major developmental stages of the central nervous system, and their relative abundance correlates well with the prevalence of neural stem/progenitor cells (Capela & Temple, 2002; 2006). These data indicates that the D3/MEF has more beneficial effect to maintain stem cell competence than D3/STO and D3/–.
In conclusion, we compare the effects of MEF and STO feeder cells on mES cell culture. While no differences in colony formation and AP activity assay are observed, some pluripotency marker genes and proteins are highest detected in D3/MEF than D3/STO or D3/–. It indicates that more suitable feeder cell for mES cell culture is MEF cell. Although MEF cell has disadvantage that needs to repeatedly isolation from fetus tissue, their potential as feeder cell layer are larger than STO cell line that commercialized cell line growing permanently. The good quality ES cell colonies from culture on MEF feeder cell layer will facilitate use of derived, differentiated lineages in drug screening, disease modeling and transplantation.