INTRODUCTION
Equine chorionic gonadotropin (eCG) is a unique member of the gonadotropin family, as it can perform both luteinizing hormone (LH)- and follicle-stimulating hormone (FSH)-like activity in non-equid species as well (Chopineau et al., 2001). Members of the glycoprotein family, including CG, LH, FSH, and thyroid-stimulating hormone (TSH), comprise two non-covalently linked α- and β-subunits (Min et al., 2004; Park et al., 2010, 2017; Lee et al., 2017). The β-subunits of eCG and eLH have identical primary structures (Min et al., 1996). Thus, eCG could be an ideal model for studying the structure/function associations of gonadotropins, as it exhibits the properties of both, its pituitary and placental, counterparts (Galet et al., 2009; Park et al., 2017).
In our previous study, we produced recombinant eCG (rec-eCG) mutant proteins from CHO-K1 cells, which exhibited dual LH- and FSH-like activity in rat Leydig and granulosa cells (Min et al., 1996, 2004) and in cells expressing ratLH/CGR (rLH/CGR) and rFSHR (Park et al., 2010; Jeoung et al., 2010; Lee et al., 2017) and internalized rLH/CGR and rFSHR (Park et al., 2017).
Japanese eel is one of the most important fish species being aquacultured in East Asian countries, including Japan and Korea (Kagawa et al., 1998). Eel gonadal development can be induced by administering gonadotropin reagents, such as the salmon pituitary extract (Kim et al., 2016a; Kazeto et al., 2008; Kobayashi et al., 2010). rec-eel FSH and LH have recently been widely reported to be involved in reproductive endocrinology (Kobayashi et al., 2010; Kazeto et al., 2008; Ohta et al., 2007). We also recently analyzed the biological activities of the specific monoclonal antibodies for rec-eelFSHβ/α and the rec-eelLHβ/α produced from CHO cells by measuring the percentage of germinal vesicle breakdown in vitro (Kim et al., 2016a,b). A large quantity of high-quality milt was transported, 18–24 h after injection, from the testes to the sperm duct for storage when treated with rec-eelLH (500 eg/kg BW) (Ohta et al., 2017).
In the present study, we constructed a mammalian expression vector containing eelLHR and eelFSHR. The expression vectors were transfected into CHO-K1 cells and PathHunter CHO-K1 Parental cells; we then assessed the signal transduction activity of eelLHR and eelFSHR upon exposure to rec-eCGβ/α and native eCG.
MATERIALS AND METHODS
The expression vector, pcDNA3, was purchased from Invitrogen (San Diego, CA, USA). CHO-K1 cells were obtained from the Japanese Cancer Research Resources Bank (Tokyo, Japan). Endonucleases were purchased from Boehringer Mannheim (MA, USA) and Takara (Osaka, Japan). Polymerase chain reaction (PCR) reagents were from Takara (Japan). Ham’s F-12, CHO-S-SFM II, Geneticin, Lipofectamine 2000, and fetal bovine serum (FBS) were obtained from Gibco BRL (MD, USA). The QIAprep-Spin plasmid kit was purchased from QIAGEN Inc. (Hilden, Germany). FreeStyle MAX reagent, FreeStyle CHO expression medium, pCMV-ARMS1-PK2 expression vector, anti-myc antibody, antibiotics, and assay complete medium were purchased from Invitrogen, the PathHunter CHO-K1 β-arrestin Parental cell line was obtained from DiscoveRx (San Diego, CA, USA) and disposable spinner flasks were from Corning Incorporated (NY, USA). PMSG ELISA kit was obtained from DRG International Inc. (Mountainside, NJ). The cAMP Dynamic 2 immunoassay kit was from Cisbio Bioassay (France).
The oligonucleotides were synthesized by Green Gene Bio (Seoul, Korea). Fetal bovine serum was from Hyclone laboratories (Utah, USA). Centriplus Centrifugal Filter Devices were purchased from Amicon Bio separations (MA, USA). All other reagents used were from Sigma-Aldrich (USA) and Wako Pure Chemicals (Osaka, Japan).
cDNA encoding the tethered eCGβ/α was inserted in the pcDNA3 mammalian expression vector, as previously reported (Min et al., 2004). The epitope, myc-tag (Glu-Gln-Lys-Leu-Ile-Ser-Glu- Glu-Asp-Leu) was inserted into the tethered eCGβ/α between the first and second amino acids in the β-subunit of the mature protein (Min et al., 2004). The schematic diagrams of rec-eCGβ/α production are shown in a previous publication (Lee et al., 2017) (Fig. 1). These fragments were digested with EcoR I and Sal I and ligated into the eukaryotic expression vector, pcDNA3. The plasmids were then purified and sequenced in both directions by automated DNA sequencing to ensure that the correct mutations had been introduced (designated as pcDNA3-eCGβ/α).

The rec-eCGβ/α proteins were expressed, as described previously (Lee et al., 2017), by transfecting the expression vector into CHO-S cells using the FreeSytle MAX reagent transfection method according to the supplier’s instructions. Briefly, CHO-S cells were cultured with Free Style CHO expression medium at a density of 1×107 cells/ 30 mL for 3 days. One day prior to transfection, the cells were passaged at 536×105 cells/mL with CHO expression medium in 125 mL disposable spinner flasks. On the day of transfection, the cell density was about 1.2/1.5×106 cells/mL. Next, DNA (160 μg) was mixed gently in 1.2 mL of OptiPRO serum-free medium (SFM), and FreeStyle MAX reagent (160 μL) was also mixed gently in 1.2 mL of OptiPRO SFM. Both of the medium mixtures were incubated for 5 minutes at room temperature (RT). The solutions were then mixed, and the complex (2.4 mL) was added to each cell suspension flask. For the rec-protein assay, the culture medium was collected on day 7. Finally, the culture media were collected on day 7 after transfection and centrifuged at 15,000 rpm at 4℃ for 10 min to remove cell debris.
The supernatant was collected and concentrated by freeze-drying in an Amicon Stirred cell concentrator and stored at −20℃ until the assays were performed. Recombinant proteins were analyzed by enzyme-linked immunosorbent assay (ELISA), as previously reported (Lee et al., 2017).
The tethered rec-eCG proteins were produced in the CHO suspension cells and rec-eCG was quantified using the PMSG ELISA kit, according to the supplier’s protocol (DRG, USA).
The method for the construction of cDNAs encoding the eelLHR and eelFSHR was modified as previously reported (Kim et al., 2016a). The PCR fragments were ligated into the pcDNA3 (EcoRI-XhoI site) mammalian expressing vectors (Fig. 2). Transfection of CHO-K1 cells was performed using the liposome transfection method, as previously described (Lee et al., 2017). CHO cells were cultured in growth medium [Ham’s F-12 media containing penicillin (50 U/mL), streptomycin (50 μg/mL), glutamine (2 mM), and 10% fetal bovine serum]. CHO cells were grown at 80–90% confluence in 6–well plates. After combining the diluted DNA with Lipofectamine reagent, the mixture was incubated for 20 min. CHO cells were washed with Opti-MEM and the DNA-Lipofectamine complex was added to each well. After 5 h, CHO growth medium including 20% FBS was added to each well. Fresh growth medium was added at 24 h after transfection. The cells were then subjected to cAMP analysis, 48–72 h after transfection.
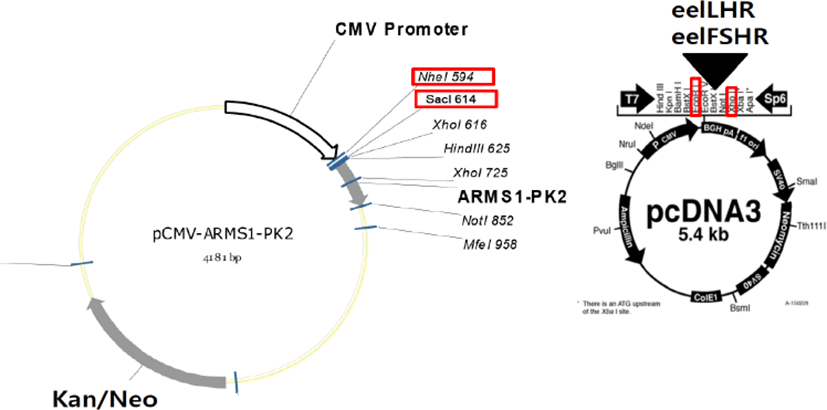
PathHunter CHO-K1 EA-Parental cells, which are engineered to stably express the enzyme acceptor-tagged β-arrestin fusion protein, were transiently and stably transfected according to the supplier’s protocol. The PCR fragments were ligated into the pCMV-ARMS1-PK2 (Nhe1-SacI site) mammalian expressing vectors (Fig. 2). PathHunter CHO-K1 EA-Parental cells were cultured in AssayComplete CHO-K1 culture medium [AssayComplete CHO-K1 medium containing 10% fetal bovine serum and antibiotics (penicillin, streptomycin, and glutamine)]. For the stable cell lines, transfected cells were seeded at 500 and 1000 cells per 100 mm culture dish at 24–48 h after transfection. The cells were cultured in AssayComplete medium containing G418 for 2–3 weeks to select for the cells expressing eelFSHR. Approximately 20 clones were recovered and cultured in a 24 well-plate. The grown cells were transferred to 6-well plates and 25 cm2 culture flasks. Finally, 5 cell clone lines were isolated and stocked, according to a previously reported method (Lee et al., 2017).
cAMP accumulation in CHO cells and PathHunter CHO- K1 EA-Parental cells was measured using cAMP Dynamics 2 competitive immunoassay kits (Cisbio Bioassays), as described previously (Lee et al., 2017). Briefly, the cAMP assay uses a cryptate-conjugated anti-cAMP monoclonal antibody and d2-labeled cAMP. The transfected eelLHR and eelFSHR cells were plated at 10,000 cells per well into 384-well plates. Five microliters of compounds in medium buffer were added to each well; then, cAMP-d2 (5 μL) and anti-cAMP-cryptate (5 μL) were added to each well. Next, the plate was read on a compatible HTRF reader. Results were calculated from the 665 nm/620 nm absorption ratio and expressed as Delta F % (cAMP inhibition). The concentration of the standard samples prepared was in the range of 0.17r712 nM (final concentration of cAMP per well).
The cAMP concentration for Delta F% value was calculated by GraphPad Prism.
Dose-response curves were fitted to a nonlinear regression variable slope equation using GraFit 5.0 (Erithacus Software Limited, Surrey, UK) and GraphPad Prism 6.0 (GraphPad Software, Inc, La Jolla, CA, USA). Curves fitted in a single experiment were normalized to the background signaling measured in mock-transfected cells (0%). The sum of each curve was calculated from at least three independent experiments.
RESULTS
After transfecting eCGβ/α vectors into CHO-S cells, the rec-eCG secreted into the serum-free medium was isolated, concentrated, and quantified using ELISA. The quantity of rec-eCG secreted was 200 mIU/mL. The molecular weight of the rec-eCGβ/α protein was found to be approximately 40–45 kDa (data not shown), which was consistent with that reported in previous studies (Jeoung et al., 2010; Lee et al., 2017).
The mammalian expression vectors for eelLH/CGR were transfected into CHO-K1 cells.
The CHO-K1 cells transfected with eelLH/CGR cDNA were counted and then the cells (10,000) were plated into 383-well plates. PathHunter CHO-K1 cells transfected with eelFSHR cDNA were isolated by G418 treatment. Finally, 10 clone cells were isolated, and the responsiveness of eelFSH receptor was analyzed by agonist treatment. The cAMP level was determined to evaluate the response induced by agonist stimulation.
The effect on cAMP stimulation in CHO-K1 cell lines expressing eelLH/CGR genes was determined to evaluate the activity of rec-eCGβ/α and native eCG. Receptor-expressing cells were incubated with various concentrations (0.085p1,500 ng/mL) of ligand. As shown in Fig. 3, Delta F% for rec-eCGβ/α (B) and native eCG (C) gradually decreased in a dose-dependent manner as compared to the standard curve (0.17s712 nM) (A). Here, the cAMP production, expressed as Delta F%, was inhibited by activation of the transfected eelLH/CGR. Next, these data were calculated by cAMP concentration (nM) as shown in Fig. 3. The cAMP concentration increased in proportion to the concentration of rec-eCGβ/α and native eCG. The EC50 values of rec-eCGβ/α and native eCG were 172.4 and 786.6 ng/mL, respectively(Table 1). The activity was higher 4.5 times for rec-eCGβ/α. Thus, the data suggest that rec-eCGβ/α and native eCG bound to eelLH/CGR and stimulated the receptors.
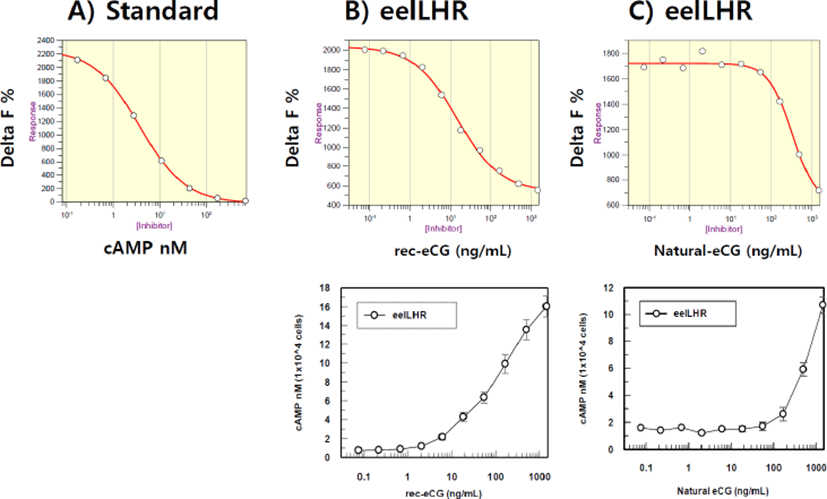
eCG type CHO-K1 cells (transient) | cAMP responses | ||
---|---|---|---|
Basal (nM / 104 cells) | EC50 (ng/mL) | Rmax (nM / 104 cells) | |
Rec-eCGβ/α | 0.53 | 172.4 (118 to 251) | 19.6 |
Native eCG | 1.54 | 786.6 (527 to 1,174) | 14.54 |
Values are the means of triplicate experiments. The EC50 values used to determine the potency were obtained from the concentration-response curves for the in vitro bioassays.
rec-eCGβ/α, recombinant equine chorionic gonadotropin ; eCG, equine chorionic gonadotropin; cAMP, cyclic adenosine monophosphate; EC, effective concentration.
First, eelFSHR plasmids were transfected into PathHunter- EA Parental cells expressing β-arrestin. Stable clones were selected by G418 treatment. Five clones of 10 positive clones were subjected to cAMP analysis by rec-eelFSHβ/α treatment. Next, we established the responsive cell lines for rec-eelFSHβ/α. As shown in Fig. 4, cAMP response to rec-eCGβ/α and native eCG was analyzed. Delta F% gradually decreased a dose-dependent manner compared to the standard curve (0.17s712 nM) (Fig. 4A). However, we did not find any dose-dependent response (0.085d1,500 ng/mL) for rec-eCGβ/α and native eCG treatment (Fig. 4 B,C). The curve was flat as shown in Fig. 4B,C. This suggests that eelFSHR does not bind with eCG. Thus, there were no changes in cAMP concentration in eelFSHR-expressing cells in response to eCG binding. eCG is, therefore, not suitable for promoting eel ovulation and maturation.
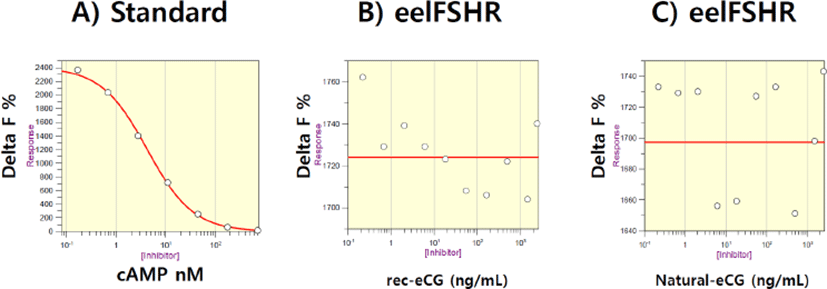
DISCUSSIONS
The results of the present study showed that rec-eCGβ/α and native eCG induced signal transduction by eelLH/ CGR in CHO-K1 cell lines that expressed these receptors in a time- and concentration-dependent manner. However, the eelFSHR-expressing cells did not exhibit any response to rec-eCGβ/α and native eCG.
In previous studies, we reported rec-eCG production in CHO-K1 cells (Min et al., 2004; Park et al., 2009, 2010; Jeoung et al., 2010). However, the specific glycosylation site is very important for the secretion of rec-eCG in CHO-K1 cells (Min et al., 2004). We also stably transfected CHO-K1 cells with rFSHR and rLH/CGR cDNA. In previous studies, rec-eCGβ/α was shown to activate both rFSHR and rLH/CGR in non-equids, but only LH-like activity was observed in equid species (Park et al., 2009; 2010). eCG does not bind to FSH receptors in equine follicles (Guillou & Combarnous, 1983; Murphy & Martinuk, 1991) or testis (Moore & Ward, 1980), suggesting that eCG is primarily an LH-like hormone in horses. However, eCG binds to donkey FSH receptors with an affinity similar to its affinity of binding to donkey LH receptors (Guillou & Combarnous, 1983; Murphy & Martinuk, 1991), a finding that has been interpreted to indicate that eCG may have FSH activity in donkey (Stewart & Allen, 1981).
One of the intriguing properties of eCG is its dual activity in species other than the horse. The analysis of the biological activity of eCG has been further complicated by the use of a variety of standards, including ovine, bovine, human and equine gonadotropins, all with different inherent activity (Aggarwal & Papkoff, 1980; Murphy & Martinuk, 1991). Our previous results agree with those of reports that state that rec-eCG exhibits biological activity (Min et al., 1996, 2004; Park et al., 2009, 2010). These results indicated that rec-eCG produced from mammalian cells could be useful for improving the development of dominant and pre-ovulation follicles in non-equids.
Labeling and pulse-chase experiments showed that the secretion of the eCGβ-subunit from CHO-K1 cells was inefficient (medium recovery of 16–25%) and slow (t1/2>6.5 h). This secretion of the eCGβ-subunit resembled that of the hLHβ-subunit, rather than that of the hCGβ-subunit (Cohen et al., 2015). Previous studies showed that rec-eCGβ/α can be expressed in the baculovirus-Sf9 insect cell system (Legardinier et al., 2008). Heterodimeric rec-eCG exhibited the same thermal stability as native pituitary LH, and rec-eCG advantages over the single-chain eCG included higher secretion, higher in vitro bioactivity, and lower risk of immunogenicity (Legardinier et al., 2008).
In the present study, rec-eCGβ/α showed higher activity than native eCG. In a previous study, rec-eCG produced from Mimic insect cells showed mammalian-like nonsialyl complex-type N-glycosylation (Legardinier et al., 2005a,b). Native eCG contains complex-type N-glycans with both Siaα2,3Gal and Siaα2,6Gal at the ends. We suggest that the differences in structure between rec-eCGβ/α and native eCG may account for the different results in the quantitative analysis by ELISA. We also suggested that the degradation rate of the mutant rec-eCG, which was deglycosylated at Asn56 of the α-subunit, was not increased because of the conformation of the hormone-receptor complex (Park et al., 2017).
Based on the data presented, rec-eCGβ/α and native eCG did not increase cAMP responsiveness in the cells expressing eelFSHR. Thus, we suggest that the dual activity of eCG differs between mammals and fish. Although eCG did not elicit any activity in the cells expressing eelFSHR, eCG increased the biological activity in the cells expressing eelLH/CHR. Therefore, we suggest that rec-eCG and native eCG are not suitable for the maturation and ovulation of female eels. We recommend that rec-eCG and native eCG can be used for sexual-maturation in female and male eels because eCG bound to the eelLH/CGR and increased the cAMP response. rec-eelFSH appears to exert biological activity in cells expressing the eFSHR gene (in preparation). Thus, we suggest that the rec-eel FSH hormone binds to the eFSHR, and eFSH may bind to eelFSHR.
Therefore, we conclude that rec-eCG and native eCG do not stimulate the cells expressing eelFSHR. The dual FSH- and LH-like activity of eCG is differ between mammalian and fish species. Further studies are required to conclusively determine whether the dual activity of eCG is indeed responsible for the uncoupling of eFSHR and eLH/CGR.