INTRODUCTION
Development of the cardiovascular system begins soon after gastrula concomitant with somite formation. In vertebrates, the cardiovascular system is the first functional organ system to arise and is critical in providing adequate oxygen and nutrient delivery to rapidly developing tissues (Chung & Ferrara, 2007; Patel-Hett & D'Amore, 2011). Cardiovascular system is very important in the developmental stages of the olive flounder life cycle. In most oviparous fish, including the olive flounder (Paralichthys olivaceus), embryo and larval survival depends upon timely vascular formation mediated by specific developmental gene expressions. Hypoxia triggers responses during developmental stage that play roles, cell to cell interaction and inhibit growth and development in fish purview from invertebrates to animals (Kajimura et al., 2006; Dunwoodie, 2009). To make sure survival in a variety of environments, fish embryos have evolved mechanisms to respond to hypoxia and repair oxygen homeostasis (Freeburg & Abrahamson, 2003). Because many embryonic tissues develop rapidly, they often outpace their vascular supply. Hypoxia-inducible genes are crucial regulatory proteins for the development of embryo to hypoxia, and they stimulate angiogenesis through activation of the vascular endothelial growth factor (VEGF) gene (Moeller et al., 2004; Liao &Johnson, 2007). Hypoxic stress response has been shown that rework the structure and mobility of endothelial cells in a way that contributes to a formation of vascular system. These mechanisms play an important role in blood vessel formation and communication with leukocytes as well as in regulation of intermediate filament in hypoxia response. The Intermediate filaments (IF) constitute one of the three major components of the cytoskeleton which also include actin microfilaments and tubulin microtubules (Goldman et al., 2012; Holthofer et al., 1984; Styers et al., 2005). Microtubules and actin filaments are dynamic structures known to play key roles in neuronal development and origin development, such as organization of neuronal cell, migration, axonal outgrowth, and intracellular transport, the functions of IF proteins in neurons have remained, until recently, more elusive (Hyder et al., 2007; Herrmann et al., 1989; Karabinos et al., 2001). The extracellular domains of desmocollin and desmoglein engage with their counterparts of adjacent cells to form the intercellular connection. In addition, in the myoplasm, intermediate filaments connect to dense bodies to which actin filaments also attach (Fuchs & Cleveland, 1998; Prahlad et al., 1998). These structures act in concert to control cell morphology and biomechanics. Vimentin is a main intermediate filament protein in airway and vascular tubule, whereas latest is expressed in smooth muscle (Qin et al., 2009) and micro arteries (Guo et al., 2005; Nekrasova et al., 2007).
The expression of intermediate filaments in flatfish developmental stages has not been sufficiently studied. In this report, we show that vascular formation causes changes in the development of olive flounder that are accompanied by changes in the expression patterns of HIF-1 and vimentin genes from fertilization to the post-hatching period. Additionally, this study examines the expression patterns of vimentin in several tissues of the olive flounder.
MATERIALS AND METHODS
All experimental fish were raised at Genetics and Breeding Research Center, National Fisheries Research and Development Institute (NFRDI) and maintained in 10 tons flow through tank at 20±1°C under a natural photoperiod. Different stages of Morula, Blastula, Gastrula, Neurula, (0.92±0.02 mm) and larvae (2.49±0.34 mm) development were described from fish kept at 20°C in the tank. Several tissues of 2 years (42 cm±SEM) old were used to in olive flounder. The samples of fish were randomly collected and frozen in 70°C deep freezer until isolation of total RNA. Several tissues were homogenized for 20 sec with Trizol reagent (Invitrogen, Carlsbad, CA, USA).
Larvae were examined as previously described, fixed at room temperature, in 2.5% glutaraldehyde (Polysciences, Inc., Warrington, PA) in 0.1 M sodium cacodylate–HCl buffer, pH 7.3, for 10 min, and post fixed in 1% paraformaldehyde (Sigma-Aldrich, CA, USA) in the same buffer, for 20 min with 1% uranyl acetate for 1 h and ethanol dehydration. The samples were examined under a stereo microscope (ZEISS CL1500 ECO Jena, Germany) imaging system at ×300 and ×500 magnification of development was determined.
Bioinformatic analysis was conducted to determine gene identities using Gene Master software (Ensoltek, Beaverton, USA). ESTs were assembled in clusters of contiguous sequences (conting) using ICA tools program (parsons, 1995). Gene annotation procedures and homology searches of the sequenced ESTs have been locally done by BLASX for amino acid similarity comparisons. Matches with the Expect value (E) less than 1.0xe–4 were considered to be significant; all ESTs were not identified as orthologs of known genes were designated as unknown EST clones.
Total RNA was extracted using the Trizol Reagent (Invitrogen, Carlsbad, CA, USA) according to the manufacturer's protocol. DNase-I (Sigma-Aldrich, CA, USA) was treated for removing genomic DNA contamination from RNA for 30 min at 37°C. The RNA samples were extracted with phenol/chloroform to inactivate the DNase I. RNA concentration was measured with spectrophotometer (Gene-Quant, Pharmacia Biotech), quality of RNA was checked by gel electrophoresis (1% agarose gel) and stored at –80°C until further use. RNA (100 ng) from each sample was transcribed to cDNA using Transcriptor First Strand cDNA synthesis kit (Roche Ltd., SWISS). The amplification was performed with AmpliTag Gold DNA Polymerase (Applied Biosystems., CA, USA) and My cycler Thermal Cycler (Bio-Rad Laboratories Inc., Hercules, CA, USA) using the following parameters: denaturation at 95°C for 10 minutes and 32 cycles of reactions of denaturation at 98°C for 10s, annealing at 60°C for 30s, and elongation at 72°C for 45s. An aliquot of each PCR product was subjected to 1.5% (w/v) agarose gel electrophoresis and visualized by staining with ethidium bromide. A search of the National Center for Biotechnology Information (NCBI) data base revealed a sequence similar to that of HIF-1, a set of expressed sequence tags (ESTs) of olive flounder, and a derived partial sequence of vimentin. The 5′ forward and 3′ reverse-complement PCR primers for amplification of each gene were as follows: HIF-1 (5'-AGTCCAAATTCATGCGGAAA-3') and (5'-GCTTTCAATTCCCTGG GTTT-3'), Vimentin (5'-CCT GCCGAACTTCTCATCTC-3') and (5'-TCCTGGAGAGT GCATG CTAA-3') β-Actin (5'-TGATGAAGCCCAGAG CAAGA-3') and (5'-CTCCATGTCATC CCAGTTGG-3'). Relative amount of each messenger RNA was quantified by dividing by density of housekeeping gene (Gene bank, HQ 386788.1).
To evaluate arrestin mRNA levels, these primers were specifically designed to detect and quantify cDNA sequences without detecting genomic DNA. The Fast-Start DNA Master SYBR Green I (Roche Ltd., SWISS) was used as fluorescent reporter dye to detect amplification products in 7500 Fast Real Time PCR System (Applied Biosystems Inc. Carlsbad, CA, USA) using the following cycling conditions: denaturation at 95°C for 10 min and 40 cycles of reactions of denaturation at 95°C for 10s, annealing at 58°C for 30s, and elongation at 72°C for 30s. Each sample was tested in triplicate to ensure statistical significance. The PCR efficiency (E%) of gene was derived from perforin (96.2%) and β-Actin (95.4%) respectively. The relative quantification of arrestin gene expression was performed using the comparative Ct method. The Ct value is defined as the point where a statistically significant increase in the fluorescence has occurred. The number of PCR cycles (Ct) required for the ROX intensities to exceed a threshold just above background was calculated for the test and reference reactions. In all experiments, β-Actin was used as the endogenous control. Results were analyzed in a relative quantitation study with the vehicle treated.
Data were analyzed using Sigma plot for Windows (Jandel Scientific, San Rafael, CA, USA). For unpaired matched comparative analysis of multiple groups, an analysis of variance (ANOVA) was performed. Data that did not meet normality assumptions were subjected to one way ANOVA on ranks, and then pair wise comparisons were made using the Student–Newman–Keuls (SNK) method.
RESULTS
To determine the origins of the vascular system of the olive flounder during the early stages of development, we used a dissecting stereo microscope to observe the lateral side of live olive flounder larvae 1 day after hatching (DAH). The most striking observation in the flounder larvae was that the beating heart was visible at 1 DAH, while the formation of blood vessels was in the developmental stage. During this period, the heart, lymph nodes, and the blood vessels all developed to their normal size and shape (Fig. 1A, B). The initial morphological changes that lead to the formation of blood vessels in olive flounder larvae were clearly visible as early as 1 DAH. The vascular system of 1-DAH larvae consists of a dorsal aorta, cardinal vein, and intersegmental vessels (Fig. 1B). At 1 DAH, the intersegmental vessels surround the notochord and the posterior cardinal vein extends laterally to connect with the dorsal aorta, which functions as the collecting system; this is illustrated in Fig. 1B. These findings demonstrate that the development of normal physiology in flounder larvae can be at least partially attributed to the development of the blood vessels.
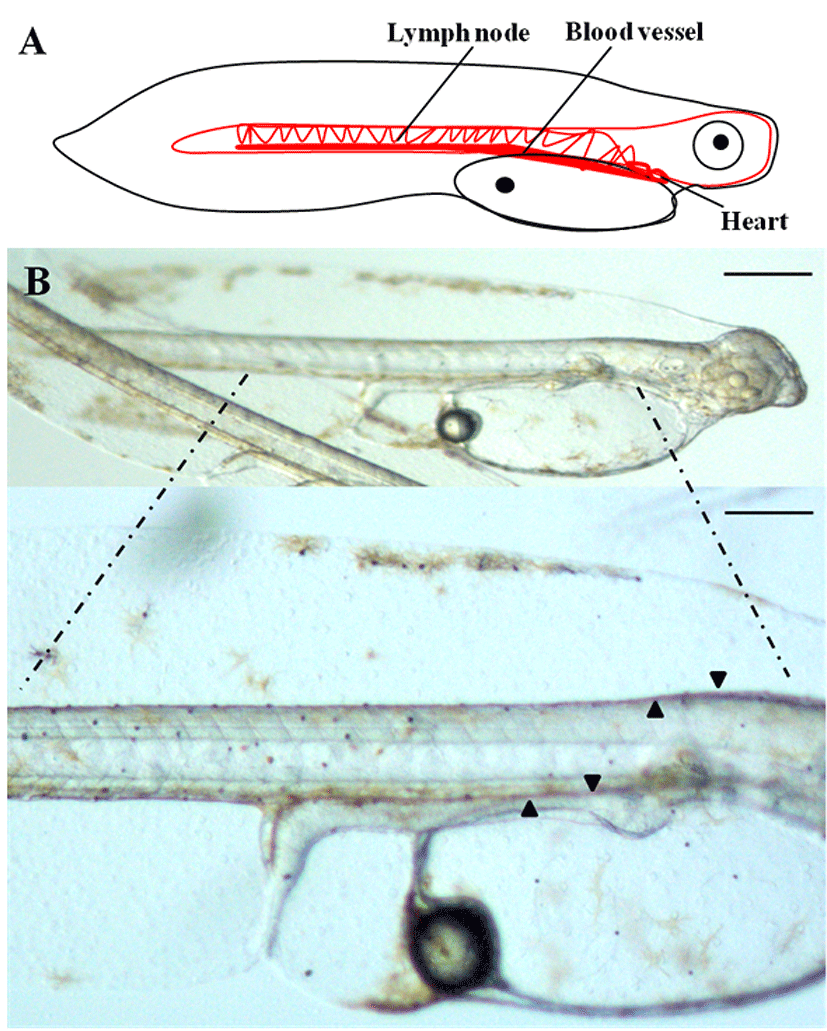
In a previous study, we constructed a cDNA library using samples from the eyes of olive flounder; 270 clones were screened against this library (Lee et al., 2009; Lee et al., 2010). Utilizing the cDNA library, several expressed sequence tags (ESTs) were found to be homologous to genes that are related to the formation of the olive flounder’s ocular structures; in addition, one EST demonstrated significant sequence similarity with the genes responsible for the expression of vimentin, a protein that plays an important role in the ocular system. A blast search of the GenBank database was performed on the homologs of the olive flounder-derived vimentin. The alignment analysis demonstrated that the amino acid sequence of olive flounder-derived vimentin is homologous to the sequences of genes that encode vimentin from other organisms, demonstrating 93–90% identity. In addition, the vimentin of the olive flounder shared the greatest level of similarity with the red seabream, tiger puffer, brichardi, Nile tilapia, cichlid, and zebra mbuna (Fig. 2A).
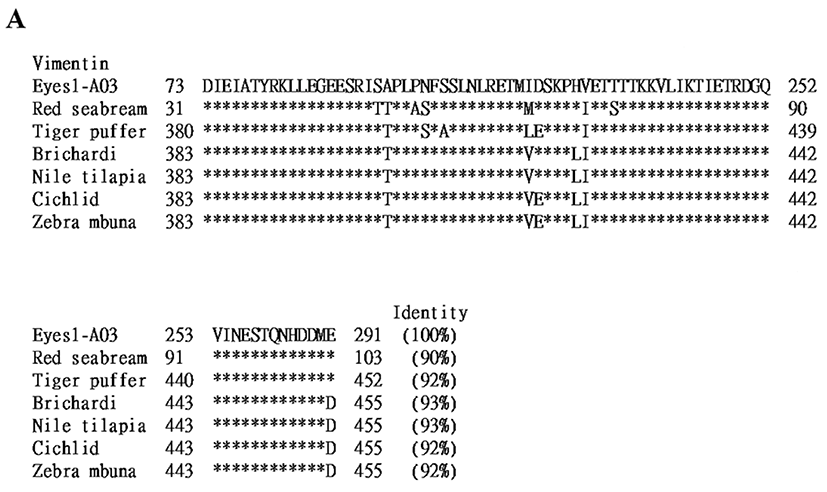
The expression of the newly identified genes encoding HIF-1 and vimentin in olive flounder embryos and larvae was detected using traditional reverse transcription-polymerase chain reaction (RT-PCR). Although the expression of the genes encoding HIF-1 was detectable post-fertilization, the expression level was found to be the greatest at 14 hour post fertilization (HPF), and decreased quickly to until 28 HPF. The expression of the genes encoding vimentin was also detectable post-fertilization, and the expression of the genes could be detected 24 HPF, with the level of expression increasing continuously up to 5 DAH (Fig. 3A). The expression of HIF-1 and vimentin in the olive flounder was examined at various stages of development by using Real-time-PCR. The expression of HIF-1 and vimentin was found similar from post-fertilization or 24 HPF to 5 DAH (Fig. 3B, C). When examining the expression patterns in the tissues of the olive flounder, vimentin mRNA was found to be expressed at similar levels in the brain, fin, eye, and spleen, while it was not expressed in the muscles, liver, kidney, and gills (Fig. 4A). The expression of vimentin was found to increase with age in tissues containing neuronal intermediate filaments (IFs), but it decreased with age in non-IF tissues (Fig. 4A, B). These results show that vimentin is expressed during the formation of the vascular system, suggesting that HIF-1 plays a role in the formation of blood vessels during the early stages of development.
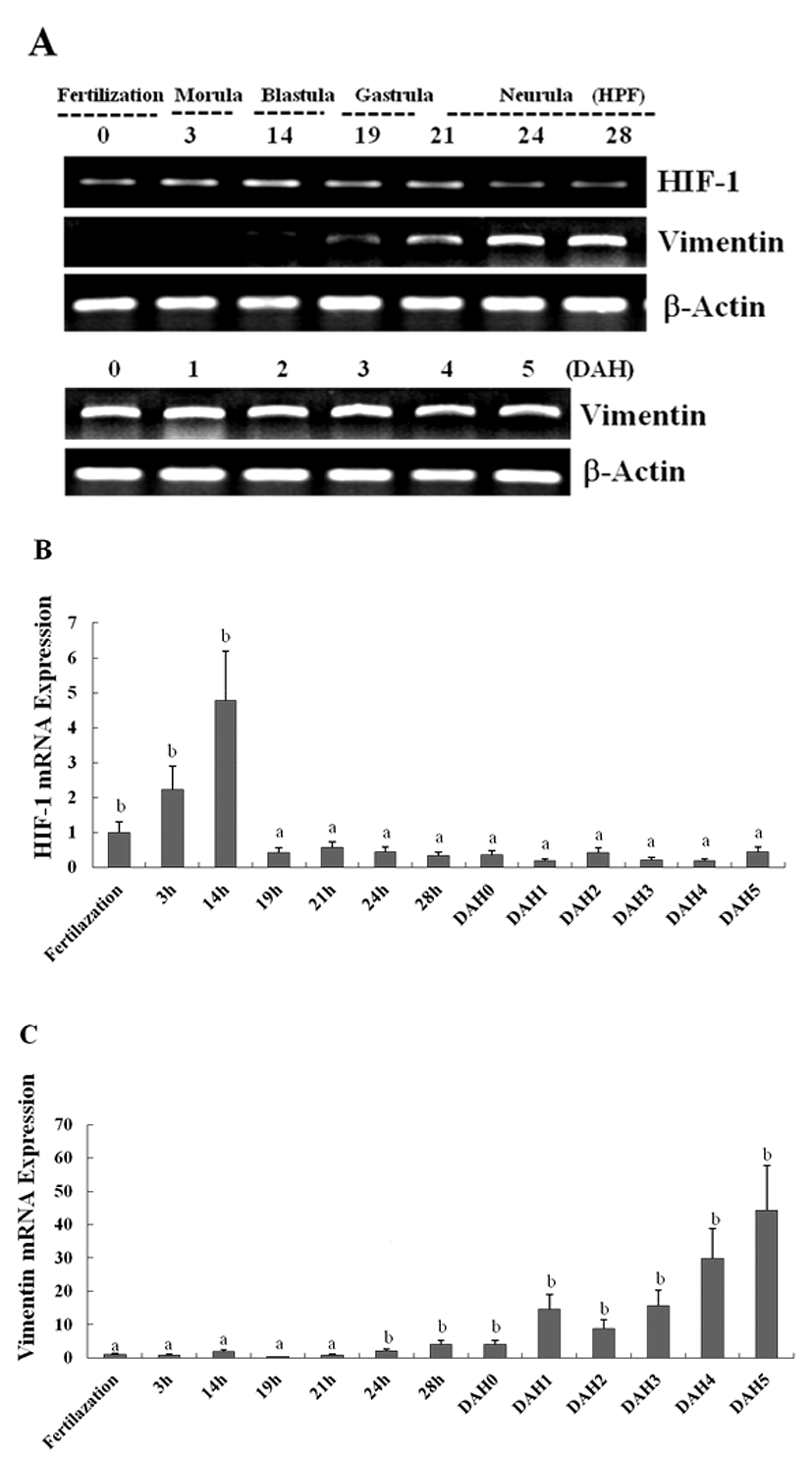
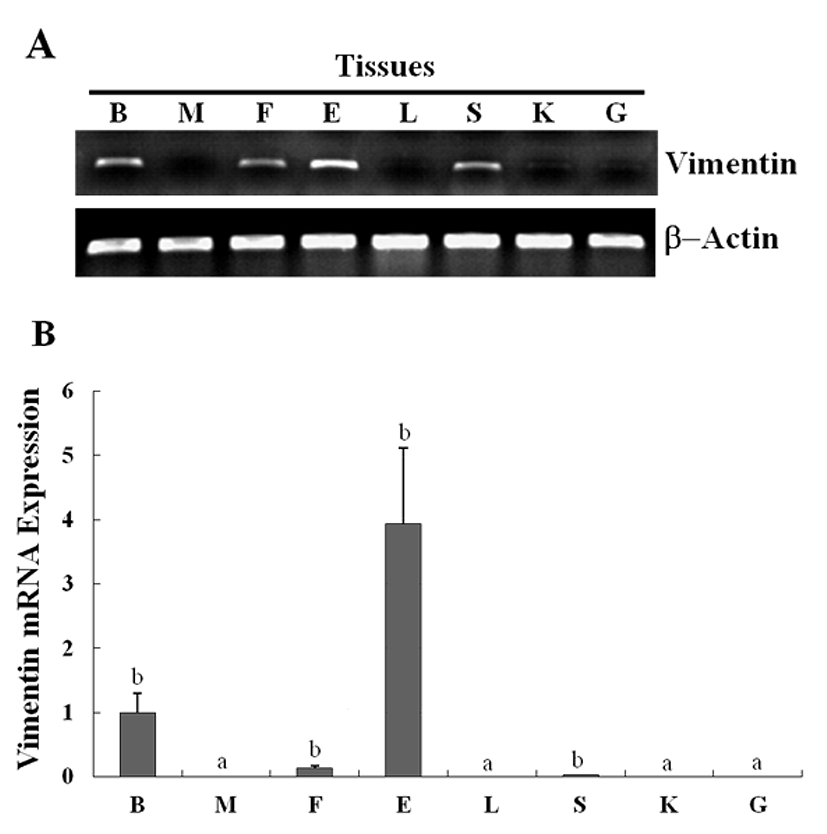
DISCUSSIONS
The changes that were observed in the expression of several development-mediating genes can be at least partially attributed to the formation of blood vessels during the early developmental stages in olive flounder larvae. At 1 DAH, it was possible to visualize the vascular system lateral to the notochord (Fig. 1B). Our results suggest that normal development of the vasculature is essential for ongoing angiogenesis. However, the formation of blood vessels in olive flounder larvae during the developmental stages has not yet been described in any study. The modulation of gene expression by cellular responses to hypoxic stress plays an essential role in early development. For example, a previous study has demonstrated that knocking down HIF-1α, HIF-2α and HIF-1β in mice (Kotch et al., 1999; Tomita et al., 2003), results in abnormal-blood-vessel development and death. In addition, knockout of HIF-1α resulted in mesenchymal cell death leading to death occurring during the middle period of gestation; this occurred owing to severe defects in cardiovascular and neuronal plate development (Grimm et al., 2002; Semenza & Prabhakar, 2007). Although HIF-1α is essential for VEGF induction during embryonic development, it is not solely responsible for VEGF activation (Okada et al., 2005). Mutations to HIF-1α do prevent endothelial cells from reaching full maturity (Manalo et al., 2005). Consistent with previous reports, we found that, in the olive flounder, HIF-1 is expressed during the early stages of development, and that vimentin is expressed during the formation of blood vessels and in the neuronal organs, lymphoid tissue, and connective tissue (Fig. 3, Fig. 4). Various studies have previously reported an increase in the development of endothelial mono layers in cell cultures in response to conditions of hypoxic stress. The key proteins of intermediate filament proteins in endothelial cells are vimentin and keratin (Tsuruta & Jones, 2003). Most blood vessels and neuronal tubes in endothelial cells are known to consist of vimentin. It has been reported that astrocytes from vimentin-knockout mice lack properly developing blood vessels and growing nerve fibers (Gariano et al., 1996). Recent studies examining the lymphocytes from vimentin-knockout models indicate that vimentin is essential for the feedback mechanisms of leukocytes and that its absence compromises the leukocyte barrier (Nieminen et al., 2006; Leong et al., 2008). RT-PCR data have demonstrated that vimentin mRNA is expressed and is detectable until 5 DAH (Fig. 3A, C). An interesting characteristic of the expression of vimentin in intermediate-filaments is the relatively high expression during blood-vessel formation and in lymphoid organs. Blood-vessel formation was observed during the early stages of developmental, and the genes encoding vimentin were highly expressed at these stages. This suggests that vimentin may play a crucial role in the development of the vascular system during the embryonic and larval stages in the olive flounder life cycle. Consequently, it is possible to conclude that vimentin is an important cytoskeleton protein and that it is a key constituent of connective tissue. Additionally, vimentin is important in the growth and maturation of the organs of the circulatory system, and plays an important role during periods of hypoxic stress.
To summarize, this analysis of the expression of vimentin during the developmental stages of the olive flounder might provide information that could be useful in understanding initial blood vessel formation and maturation. Further functional studies on this gene will help to elucidate the molecular regulatory mechanisms and the signal-transduction systems involved in the formation of the vascular system in olive flounder, as well as identifying the genes responsible for modulating the activity of vimentin.