INTRODUCTION
Hemodynamic changes are involved in the cyclic remodeling of ovarian tissue that occurs during final follicular growth, ovulation, and new corpus luteum (CL) development (Acosta et al., 2002; Brannstrom et al., 1998; Collins et al., 1991; Moor et al., 1975). Extensive vascular formation (angiogenesis) and cell differentiation (luteinization) occur in the follicular wall during the period between the luteinizing hormone (LH) surge and early CL development. At ovulation, follicles collapse and release the ovum and antral fluid. The intensity of angiogenic processes reaches a peak 2−3 days later, in early CL (Reynolds et al., 2000).
Color Doppler ultrasonography is a useful, non-invasive technique for evaluation of ovarian vascular function, allowing a visual observation of blood flow in a delimited area in the wall of preovulatory follicles or within the CL (Acosta et al., 2002; Miyazaki et al., 1998).
The regression of a cyclic CL is a very complex process, which involves major changes in tissue composition. At 24−48 h after induced luteolysis in the bovine CL, there is pronounced oligonucleosome formation with histological signs of luteal cell degeneration (Cavender & Murdoch, 1988; Juengel et al., 1995). The CL is a heterogeneous gland, which is composed of fibroblasts and immune cells, in addition to steroidogenic luteal cells and endothelial cells. The immune cells and their cytokine products are involved in the regulation of luteal function (Pate, 1994; Penny et al., 1999).
The transition from pre-ovulatory follicle to CL is a dynamic process involving a series of biochemical and morphological changes following the LH surge, which includes angiogenesis, the formation of new blood vessels from existing vessels (Reynolds & Redmer, 1999). Indeed, the rates of luteal growth and angiogenesis are such that they are only rivaled by the fastest growing tumors, and in the mature CL, the majority of luteal cells are adjacent to one or more capillaries (Reynolds & Redmer, 1999). While luteal and follicular angiogenesis are likely to be broadly similar, there are several important differences. Firstly, the breakdown of the basement membrane following ovulation enables endothelial cells and pericytes to invade and vascularize the luteinizing granulosa cells. Secondly, the timescale of luteal development is much shorter and, thus, angiogenesis is more intense. Indeed, up to 85% of the proliferating cells in the developing CL are thought to be related to vascular remodeling, since there is extensive intermingling of all cell types (Stocco et al., 2007). The extensive vascularization of the CL enables it to receive one of the highest blood flows per unit tissue mass. Luteal blood flow remains at pre-ovulation levels in the collapsed follicle, but thereafter gradually increases in parallel with increases in luteal volume and coincides with increases in progesterone (Acosta et al., 2002). Angiogenic factors produced by the CL of cows, pigs, and sheep are primarily heparin binding and can be neutralized with antibodies against fibroblast growth factor (FGF), vascular endothelial growth factor (VEGF), and tumor necrosis factor (TNF). Angiogenesis involves a complex series of cellular processes and molecular changes. In adults, it is largely limited to pathological situations such as tumor growth and wound healing. However, the ovary undergoes continual cyclical changes and, thus, requires continual angiogenesis (Fraser & Lunn, 2001; Reynolds & Redmer, 1999). VEGF is a potent mitogen for endothelial cells and a stimulator of vascular permeability. These biological activities are important in the cascade of events leading to angiogenesis, which is essential for the developing follicle and CL. In addition, VEGF is the most important factor in the regulation of normal and abnormal angiogenesis.
TNF, a nonglycosylated protein with a molecular mass of 17 kDa, first described as a tumoricidal factor produced by activated macrophages, has a lower concentration in the bovine endometrium during the early luteal stage than at other luteal stages (Miyamoto et al., 2000). Extensive research during the last decade suggests that TNF plays one or more physiological roles in the CL in a variety of species. It has been demonstrated that TNF inhibits gonadotropin-supported progesterone production by murine, porcine, and bovine luteal cells and stimulates prostaglandin synthesis by bovine luteal cells. These findings imply that the actions of TNF on CL function are concerned with luteal regression. Indeed, the interaction of TNF and inflammatory cells is postulated to promote the regression of the CL (Bagavandoss et al., 1988). Hence, if TNF has one or more roles in luteolysis, functional TNF receptors (TNFRs) should be present in the CL, at least during luteal regression.
The identification of genes expressed within the cells of a given tissue is a basic step in the determination of gene function and the analysis of tissue physiology. One efficient approach to the characterization of gene transcripts is the expressed sequence tag (EST). ESTs are generated by largescale sequencing of randomly selected clones from cDNA libraries, constructed from mRNA. While the functions of many genes can be deduced from their homology to known genes in databases, the functions of most emerging genes and regulatory sequences are unknown. Sequence information from ESTs can be used in many other applications, such as the generation of physical maps of chromosomes, the development of genetic markers by microsatellite tagging, identification of single nucleotide polymorphisms, and development of microarrays for functional genomics (Murakawa et al., 1994; Serapion et al., 2004). The Basic Local Alignment Search Tool (BLAST) is a well-established software program in bioinformatics research used to compare a sequence against libraries of known sequences to determine sequence similarity. A snapshot of genes expressed in a given tissue and at a given developmental stage. Therefore, ESTs provide valuable information for functional genomics and bioinformatics research. Although major database (DB) websites, including NCBI, provide BLAST services and EST data, a local DB search system is needed for better performance and security.
Here, we present animal EST DBs and a local BLAST search system. The EST DBs in NCBI Genbank were divided by animal species using the perl script which we developed. We also built extended DB search systems for new data (Local animal BLAST search system), which was constructed on a high capacity PC cluster system for better performance.
MATERIALS AND METHODS
Corpora lutea were removed transvaginally from regularly cycling dairy cows with physiological saline (PBS), cut open along their longitudinal axes, and processed according to the protocols described below. The CL were cut into 4 pieces, 3 of which were immediately snap frozen in liquid nitrogen, transported to the laboratory, and stored at −80°C until RNA extraction was performed. In the first experiment, CL’s were removed at 5 stages of the estrous cycle; corpora hemorrhagic CH2 (1−2 cm), CH3 (>2 cm), CL (1−2 cm), CL3 (>2 cm).
Frozen tissue collected for the first experiment was homogenized in TRIzol (Brinkman instruments, Westbury, NY) reagent using a polytron tissue homogenizer. In the second experiment, RNA from cultured cells was isolated by adding TRIzol reagent directly to culture flasks. Following tissue homogenization or cell lysis, total cellular RNA was isolated according to procedures outlined by the manufacturer. The final RNA precipitate was resuspended in double-distilled water containing 0.1% diethyl pyrocarbonate (DEPC), and the RNA concentration was determined using an ultraviolet spectrophotometer at 260- and 280-nm absorbance. The A260:A280 ratio was >1.8 for all RNA samples. The RNA integrity was assessed by examining the 28S and 18S ribosomal RNA bands on ethidium bromide stained agarose formaldehyde gels. The samples were stored at −80°C until use.
Semi-quantitative reverse transcription-polymerase chain reaction (RT-PCR) was carried out with the housekeeping gene, lyceraldehydes 3-phosphate dehydrogenase (GAPDH), as an internal standard. GAPDH is constitutively expressed and has been used effectively in studies on the regulation of gene expression in ovarian cells (Chomczynski & Sacchi, 1987; Spanel-Borowski & van der Bosch, 1990; Tsai, Wiltbank, & Bodensteiner, 1996). The RT-PCR amplification was calibrated to determine the optimal number of cycles to allow detection of transcripts while keeping the amplification of these genes in the log phase (primer dropping method). GAPDH oligonucleotide primer pair (sense 5′- CAATGAACCCCTTCATTGACC-3′; antisense 5′-AGCA CCTCCCTGAATACTGG-3′) and the EST gene oligonucleotide primer pair (sense 5′-CTCAGCCCAACATGA GGATA-3′; antisense 5′-TTTCCCAGGACTGCTCCTTT- 3′) were synthesized according to bovine sequences. The TNFR1 primer pairs (sense 5′-CACCACCACCATCTGC TT-3′; antisense 5′-TCTGAACTGGGGTGCAGA-3′) and the VEGF primer pairs (sense 5′-CACCACCACCATCT GCTT-3′; antisense 5′-TCTGAACTGGGGTGCAGA-3′) were synthesized according to bovine cDNA for TNFR1 and VEGF. The expected PCR product lengths were 452 base pairs (bp) for GAPDH, 117 bp for the ESTs, 257 bp for TNFR1, and 117 bp for VEGF. With the exception of CL, 35 cycles of PCR were conducted for GAPDH, ESTs, TNFR1, and VEGF.
The PCR amplified products of selected individual lines of three independent from wild type, mutant, and transformed strains of Haemophilus influenzae and digested to completion with PvuII. After agarose gel electrophoresis, Southern blot analysis with CL cDNA was performed as described elsewhere (Chomczynski & Sacchi, 1987).
Immunohistochemistry of apoptosis was performed on 5 μm tissue sections mounted on silanized slides. Briefly, paraffin sections were dewaxed with a xylene substitute (Polyclear solvent; Polysciences, PA, USA) and rehydrated in a graded series of ethanol. Antigen retrieval was performed by heating at 95°C in 10 mM sodium citrate (pH 6.0). Endogenous peroxidases were quenched with 0.3% hydrogen peroxide in methanol for 5 min at room temperature. After 3 times washed in 1× PBS buffer, the sections were blocked with 1% goat and 3% horse serum for 1 hr at room temperature. Sections were exposed to anti-rabbit caspase 3 polyclonal antibodies (diluted 1:100; Cat No. ab4051; Abcam) in blocking solution overnight at 4°C. Sections were washed and incubated with anti-rabbit secondary antibodies (diluted 1:300) for 1 hr at room temperature, and then rinsed and incubated with ABC detection (Vector) for 10 min. Diaminobenzidine (DAB) was used as a substrate for HRP. Sections were counterstained with PAS reagent and Harris hematoxylin containing 4% acetic acid. Tissues were dehydrated, cleared, and coverslipped using Permount (Fisher, PA, USA).
RESULTS
BLAST analysis of EST genes in day 5 CL (Fig. 1, 2) identified a bovine microtubule gene. Representative examples of RT-PCR-amplified ESTs, VEGF, and TNFR1 are shown in Fig. 3. There are distinct differences in receptor expression during early, middle, and late (days 13–18) luteal phases. The analysis of EST mRNAs is shown in Fig. 3. VEGF expression was high at the middle luteal stage (CL3) and significantly decreased during the late stage. The expression of TNFR1 was very low during the activation of ESTs and VEGF. However, its expression gradually increased during the degradation of CL2–CL1 (Fig. 4, 5). Bovine CL at various stages were used for IHC (Immunohistochemistry) (Fig. 6), semi-quantitative (SQ)-PCR (Fig. 3), real-time PCR (Fig. 4), and Southern Blot hybridization (Fig. 5). We also analyzed the expression pattern of the relationship between the ESTs and VEGF (a growth factor), and TNFR1 (cell death-related). ESTs and VEGF showed a similar expression pattern, suggesting that the appearance of ESTs might affect the expression pattern of VEGF. Staining of the CL tissue with IHC (Fig. 6) from CL formation to regression showed low cytosol and aggregation of the nucleus. During the estrous cycle, the cytosol was magnified and differentiation of the nucleus was clearly manifested. At this time, we noted large granulosa cells death and blood vessel formation. We surmised that ESTs affect the cell, and that the relationship of VEGF and TNFR1 plays a pivotal role in cell nutrition and activation, depending on the stage of CL in the bovine ovary.
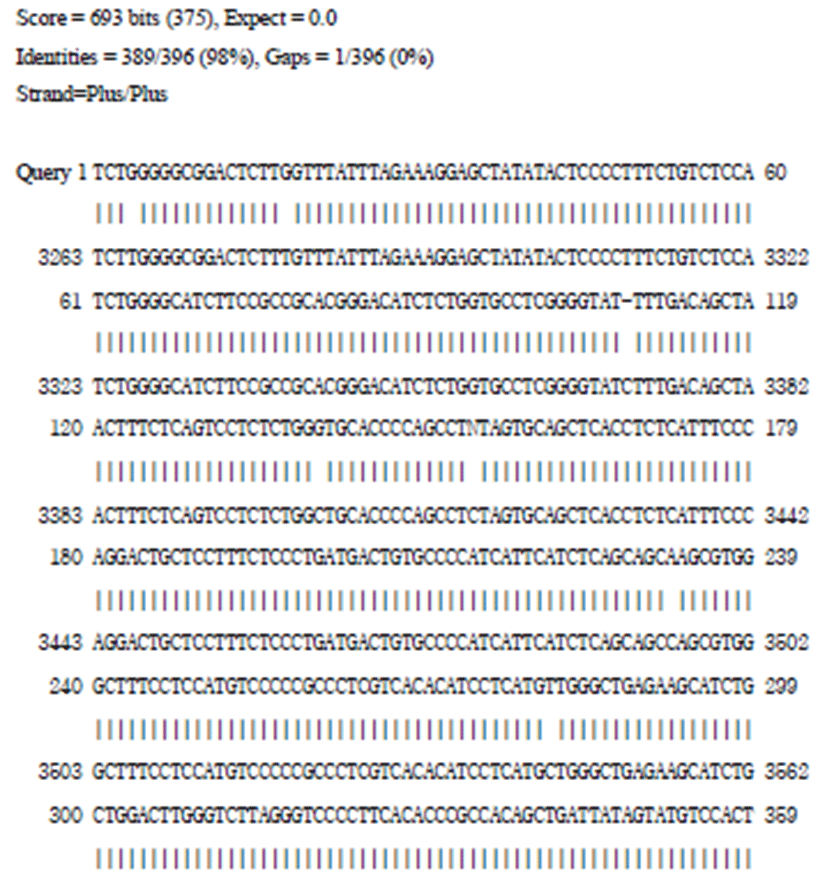
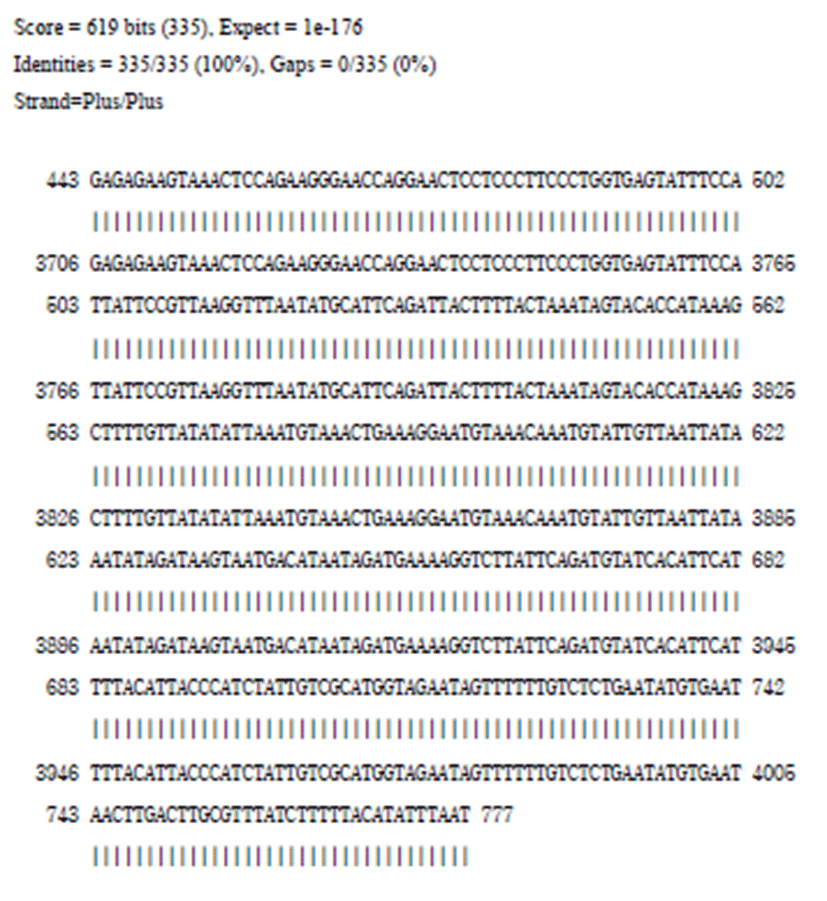
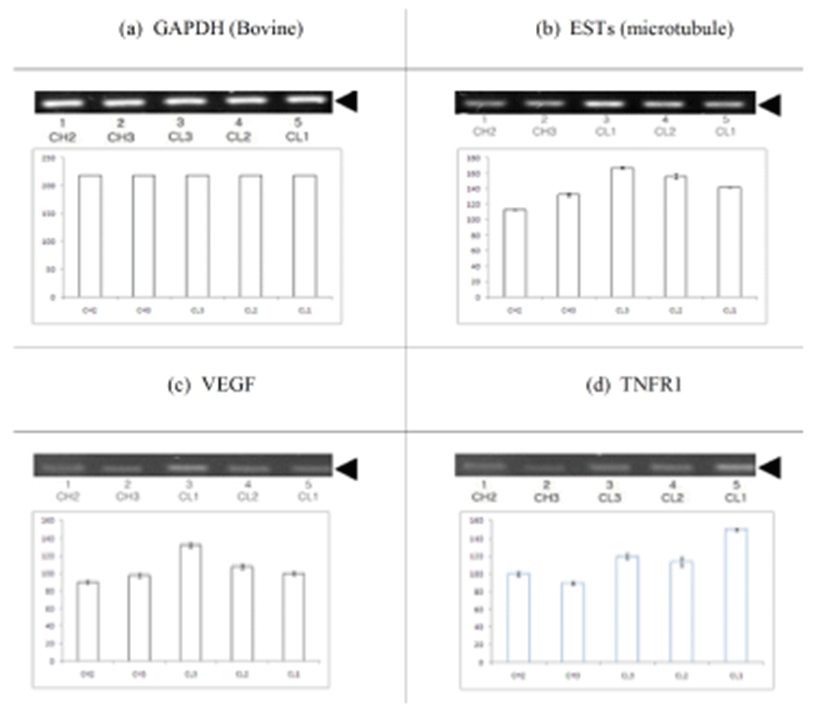
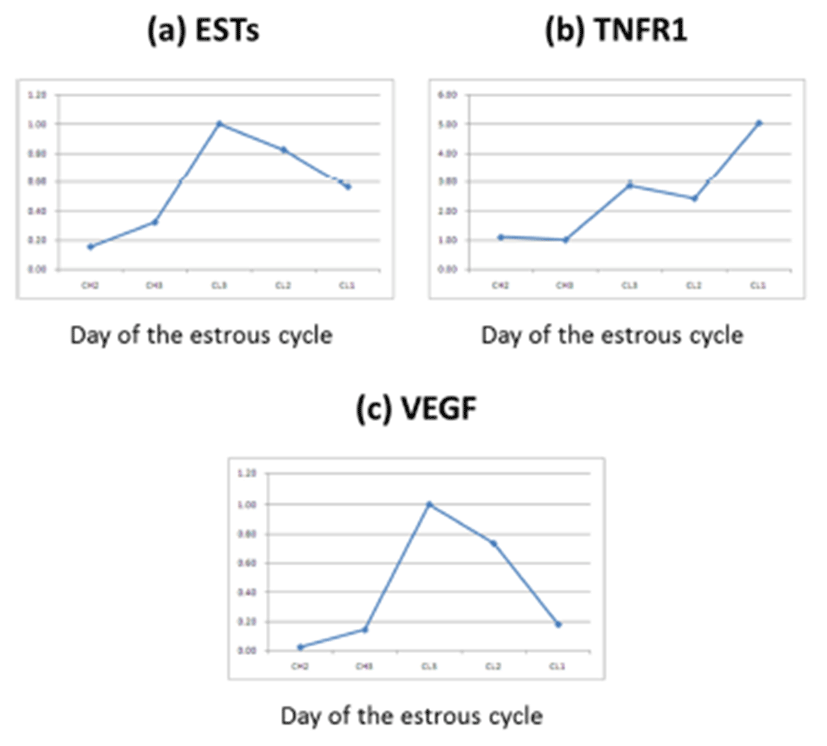
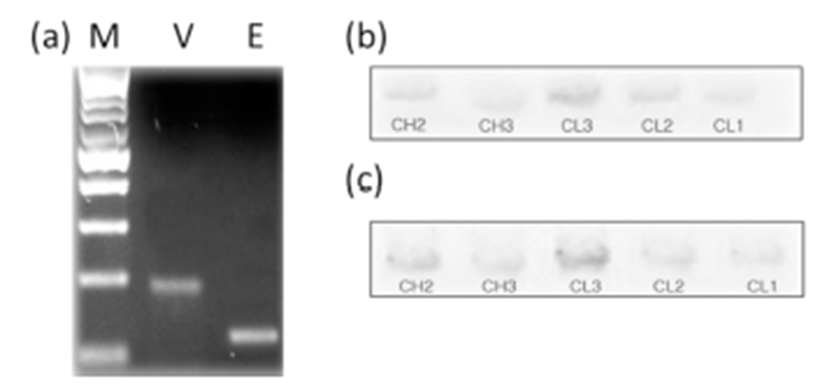
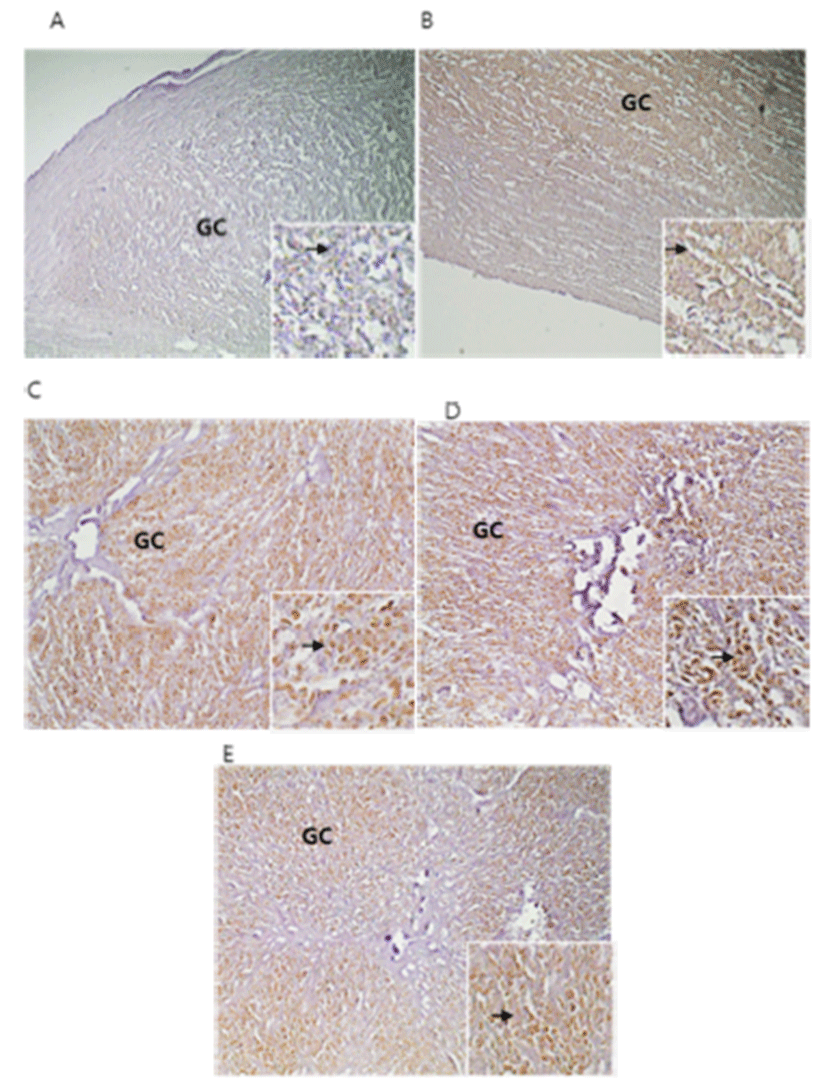
DISCUSSION
To the best of our knowledge, this study is the first to report on the expression of ESTs, TNFR1, and VEGF in the luteal tissue of any species. LH is one of the most important hormones secreted by the pituitary gland for normal luteal development and its function has been demonstrated in many species. For continuity, much of the following discussion focuses on the role of the pituitary in regulating luteal function in ewes. If the pituitary is removed on day 3 of the ovine estrous cycle, the CL does not continue to increase in weight, and serum concentrations of progesterone remain at or below levels observed on day 5 (Farin et al., 1990; Juengel et al., 1995). This loss of luteal weight, when compared with that in intact control ewes, is associated with a decrease in the number of small luteal cells (SLCs) and fibroblasts and a decrease in the size of both small and large steroidogenic luteal cells (Farin et al., 1990).
The gonadotropic regulation of the expression of mRNA for VEGF in the primate CL was studied in vivo by treating monkeys with a potent gonadotropin-releasing hormone (GnRH) antagonist during the mid-luteal phase of the menstrual cycle. A 3-day treatment regimen brought about a significant reduction in the levels of VEGF mRNA. In rats treated with human chorionic gonadotropin (hCG), increases in VEGF transcripts were detectable both in granulosa cells and thecal stromal tissue and the early CL. Cell differentiation itself plays an important role in the regulation of stimuli for VEGF expression. VEGF enables quiescent and resting endothelial cells to degrade underlying extracellular matrix, to invade and directionally migrate toward the angiogenic stimulus, and to proliferate and organize into new, 3-dimensional capillaries.
The body of evidence implicating immune cells as regulators of luteal function is expanding. Macrophages and T lymphocytes are found in the CL of a number of species, as are the mRNAs and proteins of several T cellderived cytokines (Penny et al., 1999). T cell cytokines such as interlukin-1β (IL-1β), the TNF family, and interferon- γ (IFN-γ) inhibit LH-stimulated steroidogenesis and induce prostaglandin (PGF2α) production in cultures of mixed luteal parenchymal cells. The cDNA sequence of the bovine TNF-α receptor has been reported only for the type 1 receptor. Therefore, the expression of mRNA for the type- 1 receptor in the bovine CL was examined by use of RTPCR in the present study. The mRNA of TNFR1 was expressed in the bovine CL at all stages during the estrous cycle. The concentration of TNF in the bovine endometrium at the early luteal stage was lower than concentrations at other luteal stages (Miyamoto et al., 2000). Local secretion of TNF-α in the bovine CL was higher at the late stage than during the mid-stage. TNF-α concentration in the CL were dramatically increased from levels of TNF-α mRNA expression and maintained at the same level in the CL throughout the estrous cycle. In conclusion, the overall results of the present study indicate the production and presence of local ESTs as well as functional VEGF in the bovine CL during the estrous cycle, and suggest that ESTs play physiological roles in regulating bovine CL function at the time of luteal regression as well as throughout all luteal phases. Further functional analysis of the EST genes will be required to predict their role in the CL.