INTRODUCTION
Most fish undergo the period of starvation because of wintering, spawning, migration, or regional decrease in food resources (Mustafa & Mittal, 1982; Park et al., 2001, 2015). Fish can overcome starvation through biochemical, physiological, and behavioral strategies. Endogenous energy from basic metabolic accumulation in the body is spent as fish consume their own tissues to remain alive during starvation (Mustafa & Mittal, 1982; Weatherley & Gill, 1987; Kim et al., 2012; Park et al., 2013). During starvation, essential processes in fish are maintained at the expense of accumulated (i.e., completely endogenous) energy reserves, resulting in the progressive depletion and wastage (degrowth) of body tissues (Weatherley & Gill, 1987; Park et al., 2015). The observed incidence of starvation is fundamentally common based on morphological and histological criteria (Park et al., 2001).
The efficiency of oxygen consumption in fish is directly related to their metabolic processes, through which they synthesize body ingredients to produce physical energy. This, in turn, ultimately determines their population density, food supply, and fish yield (Mehner & Wieser, 1994). Mehner and Wieser (1994) observed the relationship between the depletion of energy reserves and changes in oxygen consumption in the perch, Perca fluviatilis. Starvation reduces oxygen consumption efficiency as reported in perch larvae and the traira, Hoplias malabaricus, when they were subjected to extended periods of starvation (Mehner & Wieser, 1994; Rios et al., 2002).
Stresses on fish induce the release of catecholamine and cortisol, which cause the rapid metabolism of high-energy storage compounds (Barton & Iwama, 1991). These catabolic processes have harmful biochemical effects on fish health and lead to slow growth (Specker & Schreck, 1980). Fish exhibit primary, secondary, and tertiary responses to stress (Barton & Iwama, 1991). The primary response is manifested in rapid changes in plasma catecholamine and corticosteroid. When these responses to stressful conditions exceed normal levels, harmful secondary and tertiary responses occur. Therefore, stress induces changes in the energetic metabolic processes, reduces growth rate, disturbs reproduction, and leads to rapid changes in flesh quality after death (Barton & Iwama, 1991). Blood chemistry serves as an indicator of an animal's physiological state and many factors (age, sex, nutrition, season, and circadian rhythm) may affect the blood chemistry. Information is available from the plasma glucose changes during starvation in the Atlantic cod, Gadus morhua L., the European eel, Anguilla anguilla (Larsson & Lewander, 1973), the pike, Esox lucius (Ince & Thorpe, 1976), the toadfish, Opsanus tau (Tashima & Cahill, 1968), the goldfish, Carassius auratus (Chavin & Young, 1970), and the American eel, Anguilla rostrata (Moon, 1983). Studies of plasma free fatty acids during starvation stress have also been performed in the European eel (Larsson & Lewander, 1973), the American eel (Moon, 1983), the rainbow trout, Oncorhynchus mykiss (Sumpter et al., 1991), the pike (Ince & Thorpe, 1976), and the toadfish (Tashima & Cahill, 1968). Rios et al. (2002) noted that erythrocyte senescence and hematological changes are induced by starvation in Hoplias malabaricus.
The Far Eastern catfish, Silurus asotus (Linnaeus) (order Siluriformes, family Siluridae), spreading widely throughout the Northeast Asia, is an important species in Korean freshwater aquaculture (Kim et al., 2001; Gil et al., 2017). In this study, we tried to provide a more detailed contents, and investigated the impact of sodium nitrite on physiological response of Far Eastern catfish.
MATERIALS AND METHODS
The Far Eastern catfish, Silurus asotus used in this experiment was hatched in April 2015 and reared at Fishery Genetics and Breeding Sciences Laboratory of the Korea Maritime and Ocean University in Busan, Korea. Three experimental groups were established: initial, fed, and starved. All fish were fed twice daily with commercial feed (Table 1) in total 1%–3% food of their average body weight (BW) for 2 weeks prior to initiation of experiment in March 2016. Before the starvation experiment, fish were weighed to the nearest 0.01 g using an electronic balance (JW-1, Acom, Pochen, Korea), and their body length (BL) was measured to the nearest 0.01 cm using digital Vernier calipers (CD-20CP, Mitytoyo, Kawasaki, Japan). The fed group had an average body weight (BW) of 354.1±7.74 g and average BL of 18.2±2.79 cm (n=60). The starved group had an average BW of 356.1±9.71 g and average BL of 18.1±2.31 cm (n=60).
Nutrition | Content (%) |
---|---|
Crude protein | 40.0 |
Crude fat | 4.0 |
Crude fiber | 5.0 |
Ash | 15.0 |
Calcium | 1.0 |
Phosphorus | 1.0 |
Mineral premix2) | 1.0 |
Vitamin premix3) | 1.0 |
2) Vitamin premix contained the following amount which were diluted in cellulose (g kg–1 mix): L-ascorbic acid, 121.2; DL-α-tocopheryl acetate, 18.8; thiamin hydrochloride, 2.7; riboflavin, 9.1; pyridoxine hydrochloride, 1.8; niacin, 36.4; Ca-D-pantothenate, 12.7; myo-inositol, 181.8; D-biotin, 0.27; folic acid, 0.68; p-aminobenzoic acid, 18.2; menadione, 1.8; retinyl acetate, 0.73; cholecalciferol, 0.003; cyanocobalamin, 0.003.
3) Mineral premix contained the following ingredients (g kg–1 premix): NaCl, 43.3; MgSO4·7H2O, 136.5; NaH2PO4·2H2O, 86.9; KH2PO4, 239.0; CaH4(PO4)·2H2O, 135.3; ferric citrate, 29.6; ZnSO4·7H2O, 21.9; Ca-lactate, 304.0; CuCl, 0.2; AlCl3·6H2O, 0.15; KI, 0.15; Na2Se2O3, 0.01; MnSO4·H2O, 2.0; CoCl2·6H2O, 1.0.
Fish were reared in a recirculating system. 20 fish were placed each in 1.1-ton fiberglass-reinforced plastic circular tank (118 cm diameter, 100 cm depth); each experimental group consisted of three tanks of fish. Light was provided with four 40-W (5,400 K) fluorescent bulbs controlled by an electric timer, which kept the photoperiod at a 12L:12D cycle. Water temperature was controlled automatically and held at 25±0.5° during the experimental period. During the experiment, the fed group was hand-fed two times daily (first feeding occurred between 10:00 and 11:00 and the second between 18:00 and 19:00). The starved group was starved until the end of the starvation experiment. The fed group was given feed continuously. The fish in all experiments were kept until the starved group lost vitality completely. The survival rate during the starvation experiment was calculated retroactively, with the dead fish counted every day. The aggregate survival rates of the fed group and starved group during the experimental period were measured for each of the two triplicate groups. The acute and sublethal sodium nitrite (NaNO2) toxicity tests were conducted over period of 96 hrs. Immediately after conclusion of the starvation experiment, for the acute and NaNO2 toxiciy tests, twenty individuals (BW: 83.3±7.26 g; BL: 16.5±1.03 cm) were placed in a 150-L fiberglass-reinforced plastic tank containing 150 L of freshwater (Table 2). Nitrite (Sigma, St. Louis, MO, USA) was added to the tank to create nitrite concentration of 40–200 mg/L at intervals of 40 mg/L; nitrite-free treatment served as a control.
1) Temperature, pH, dissolved oxygen, and salinity were measured using an oxygen measurement electrode and a multi-data logger system (Oxyguard, Denmark). Ammonia, nitric acid, nitrous acid, and conductivity were measured using spectrophotometer (DR 2800, HACH, Loveland, Colorado, USA). The values are means of triplicate groups.
Three replications were used for each test concentration and control. Determining lethal concentration of fifty (LC50) values and other related statistical analysis was based upon the method described by Peltier (1978).
Immediately after conclusion of the starvation experiment, the oxygen consumption rate was measured according to the method of Jo & Kim (1999). For the measurement of oxygen consumption rate, the respirometer chamber was utilized by a simple circulating system. The water was circulated from the reservoir (170 L) to the head tank by a circulating pump, passing by a respirometer chamber and then flowing back into the reservoir. The head tank was equipped with a temperature controller and 10 µm and 3 µm cartridge filters equipped for the filtering of particles. The flow through UV lamp was utilized for the reduction of oxygen consumption by microbes. Water from the respirometer chamber flowed into an oxygen measurement chamber. During the period of experiment, the average water flow was 59.6±0.5 L/h. The respirometer chamber was comprised of an acrylic resin box with a thickness of 8 mm; the overall dimensions of the box were 10 cm (width)× 25 cm (length)×10 cm (height). A rubber pad was used as a cover for the respirometer chamber to prevent the inflow of air; a hole was made in the cover and a small valve was attached for the removal of air in the respirometer chamber. Inflow water in the respirometer chamber was diffused through a 10 mm pipe with the cap at the end, where a few holes were made. Water flowing from the respirometer chamber was flowed into the dissolved oxygen measurement chamber, the dimensions of which were 10 cm (width)× 10 cm (length)×6 cm (height). The respirometer chamber consisted of three chambers, and each chamber was connected to a dissolved oxygen measurement chamber.
Dissolved oxygen measurement chambers were equipped with an oxygen probe and air in this chamber was removed by the same method as the respirometer chamber. Dissolved oxygen was measured using an oxygen measurement electrode and a multi-data logger system (Oxyguard, Denmark). Inflow and outflow dissolved oxygen of the respirometer chamber was measured by using μLog VL 100 software at 5-minute interval over 24 hrs. Measurements of oxygen and oxygen consumption rates at each temperature were saved by the multi-data logger. After experiment started, measurement times were set at 6- hour interval over 48 hrs. Before measuring the dissolved oxygen, pH, ammonium (NH4+) concentration, carbon dioxide (CO2) concentration, and respiratory frequency (gill cover movement) were measured using a counter and a digital timer. NH4+ and CO2 concentrations were measured using spectrophotometer (DR 2800, HACH, Loveland, Colorado, USA). Dissolved oxygen and pH were measured using an oxygen measurement electrode and a multi-data logger system (Oxyguard, Denmark). Measurements of oxygen and oxygen consumption rates at each experimental group were saved by multi-data logger, as described by Cech (1990).
For the blood analyses of cortisol, glucose, and lactic acid, blood samples were extracted from five randomly selected fish at 0 (pre), 1, 6, 12, 24, and 48 hrs this post starvation stress test. Using syringes lined with the anticoagulant heparin blood was extracted and assayed at fixed intervals of 0, 1, 6, 12, 24, and 48 hrs from five experimental samples. Selected blood was filled into capillary tubes and analyzed after centrifuging at 200 g for 10 min. Plasma was then collected and stored in a deep freezer (SW-UF-200, Samwon Freezing Engineering, Busan, Korea) at −80° until analysis. The cortisol concentration was measured using radioimmunoassay. Cortisol was determined in 50 μL samples using RIA kits (Coat-A-Count TKCO Cortisol RIA Kit; DPC, USA). Mixtures of sample in 100 mL antiserum were incubated for 45 min at 37°, and then 1,000 mL separation reagent was added. The mixture was placed in a refrigerator at 4° for 15 min and then centrifuged at 1,200×g for 15 min. Supernatant was assayed for gamma radiation using an automatic gamma counter (Cobra, Packard, USA). Plasma glucose concentration was analyzed according to methodology of Raabo and Terkildsen (1960; Kit 510, Sigma, St Louis, MO, USA), where production of H2O2 by glucose oxidase in the presence of o-dianisidine was evaluated as an absorbance increase at 450 nm. The lactic acid concentrations were analyzed using blood automatic analysis (Boehringer Mannhein Reflotron, Germanry). Data were analyzed by one and two-way ANOVA with SPSS statistical package (SPSS 9.0, SPSS Inc., USA). The experiment was performed three times, and means were separated by using Duncan's multiple range test and they were considered significantly different at p<0.05 (Duncan, 1955).
RESULTS
After 210 days, the starved group of Far Eastern catfish, Silurus asotus rapidly lost vitality, and as a result, the experiment was terminated. Fig. 1 shows changes in survival rate during the experimental period. Accumulated survival was 92.2±0.47% in the fed group and 74.4±2.59% in the starved group in each triplicated tanks (p<0.05). Starvation resulted in retardation of growth, which has provided a good example of the reduction of the final group continuing to grow and remaining healthy (Fig. 2).
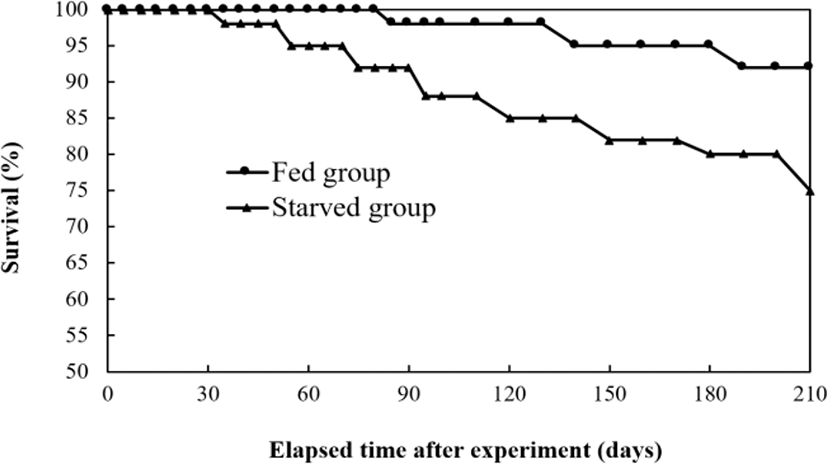
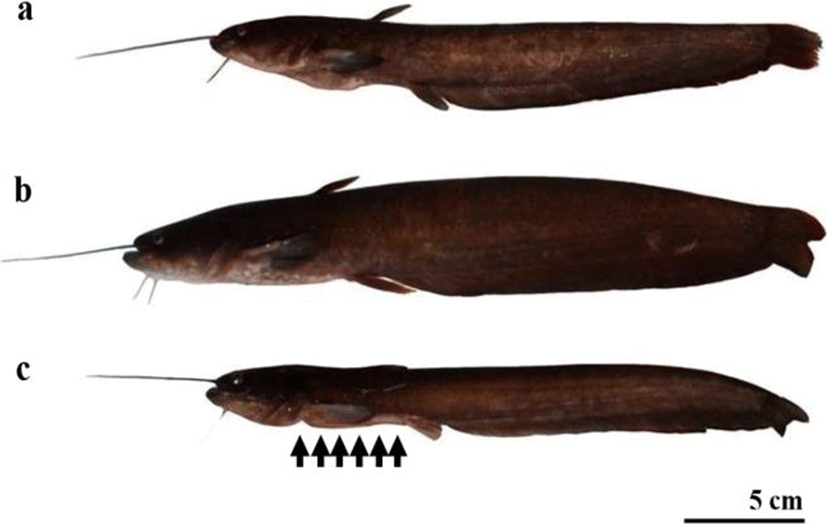
In Tables 3 and 4, and Fig. 3, the two-way ANOVA test and measurement results of oxygen consumption rate of fed and starved groups are presented. Dissolved oxygen concentration of starved groups decreased until 48 hrs, and fed groups until 16 hrs. Table 3 shows the comparison of pH values and respiratory frequency (gill cover movement) between fed and starved groups. For both groups, respiratory frequency also gradually decreased until 48 hrs and pH also decreased drastically until 12 hrs, and then de creased gradually until 48 hrs. The respiratory frequencies of starved group were significantly higher than those of fed group, and they decreased over the elapsed time (p<0.05). Therefore, it was concluded that oxygen uptake per unit of respiratory movement was lower in starved group than in fed group. The CO2 and NH4+ concentrations increased drastically until 6 hrs, and increased gradually until 48 hrs as shown in Table 4. The CO2 and NH4+ of fed group were significantly higher than those of starved group, and they increased over the elapsed time (p<0.05).
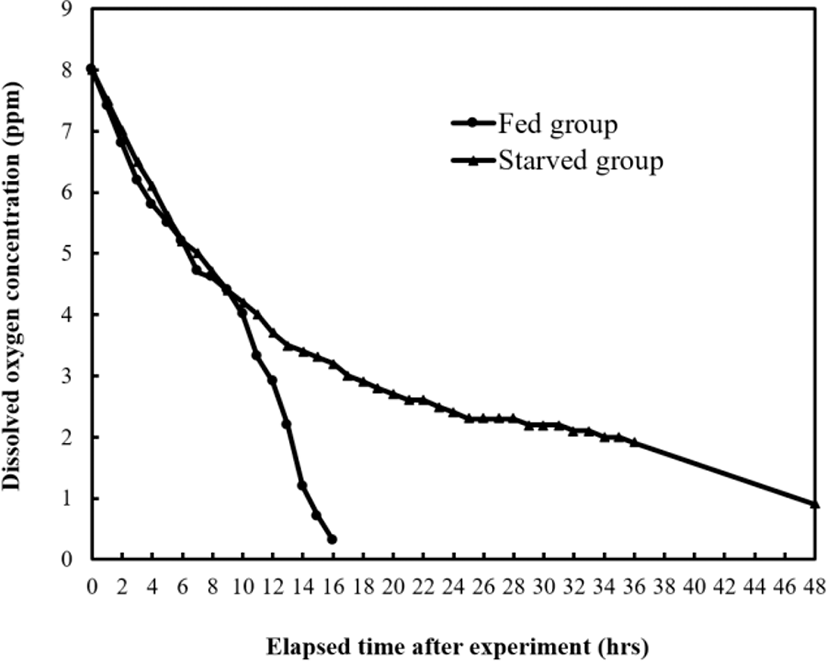
Dose–response mortality occurred in fish exposed to 120 mg/L of sodium nitrite at the fed group and 40 mg/L at the starved group (Fig. 4). Almost all fish survived 96 hrs of exposure to 0 and 40 mg/L of nitrite, whereas 75% and 25% of fish each survived 96 hrs of exposure to 150 and 200 mg/L of nitrite in fed group, and 80 and 120 mg/L of nitrite in starved group respectively. The LC50, 95% confidence ranges, and experimental conditions for different exposure groups are summarized in Table 5 and Fig. 5. The LC50 values for 96 hrs were 181 mg/L at the fed group and 108 mg/L at the starved group respectively.
Experimental group1) | 96 hrs LC50 (ppm) [95% confidence ranges] | Experimental conditions2) | |
---|---|---|---|
DO | pH | ||
Fed group | 181.53 [171.30–199.86] | 8.2±0.64 | 7.3±0.61 |
Starved group | 108.92 [81.00–123.44] | 8.1±0.41 | 7.4±0.63 |
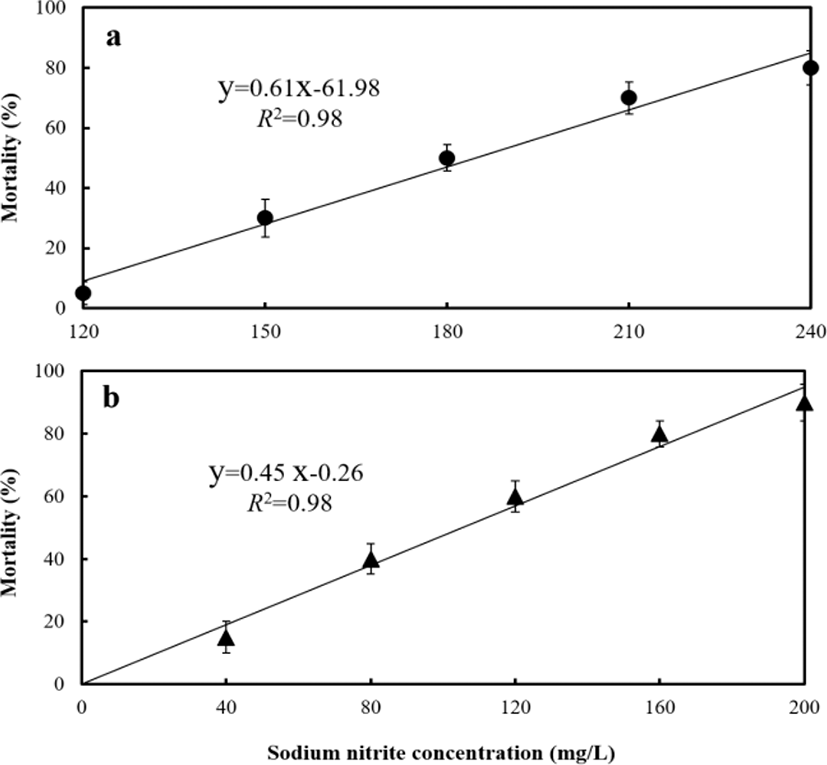
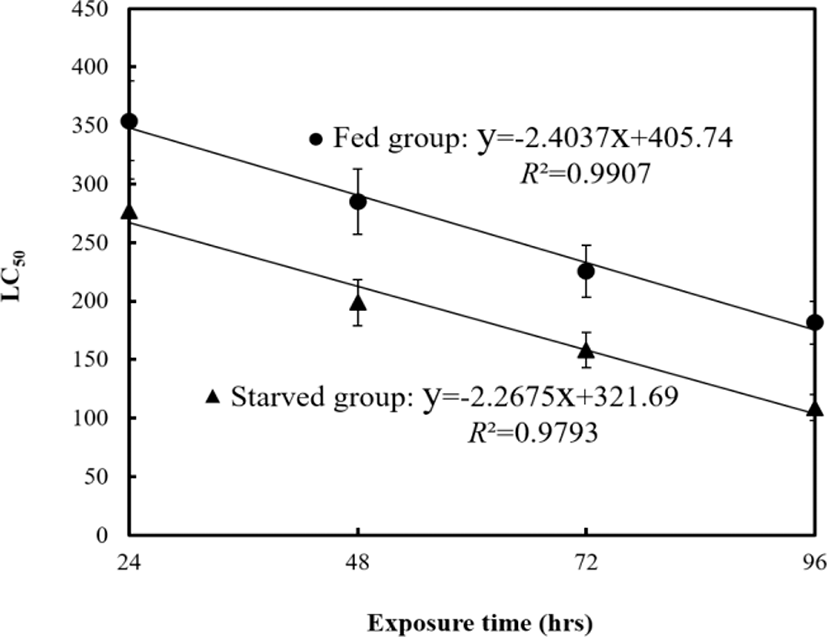
Figs. 6, 7, and 8 are variations of hematological result for 48 hrs of exposure to sublethal sodium nitrite. In the fed group, plasma cortisol increased significantly (p<0.05) from 1.9±0.3 µg/dL to 30.7±2.5 µg/dL at 12 hrs, but 5.6±2.9 µg/dL at 24 hrs and 4.8±0.7 µg/dL at 48 hrs respecticely. The changes for 24 hrs and 48 hrs were not significant (p>0.05, Fig. 6). In the starved group, plasma cortisol increased significantly (p<0.05) from 1.8±0.3 µg/dL to 16.7±2.5 µg/dL at 6 hrs, but 5.8±2.9 µg/dL at 12, 5.6±2.9 µg/dL 24 hrs, and 4.8±0.7 µg/dL 48 hrs respectively however, the changes for 12, 24, and 48 hrs were not significant (p>0.05, Fig. 6). The glucose concentrations of the two groups increased significantly from 28.5±0.7 mg/dL to 242±18.4 (fed) and 127±3.9 mg/dL (starved) at 12 hrs (p<0.05 for both, Fig. 7). The glucose levels of the fed and starved groups differed significantly from 1 hr to 48 hrs (p<0.05). At 12 hrs, the cortisol and glucose levels of the fed and starved groups reached the most significant difference (p<0.05). Lactic acid of fed and starved groups increased until 48 hrs, but no significant difference between fed group and starved group was found (p>0.05, Fig. 8).
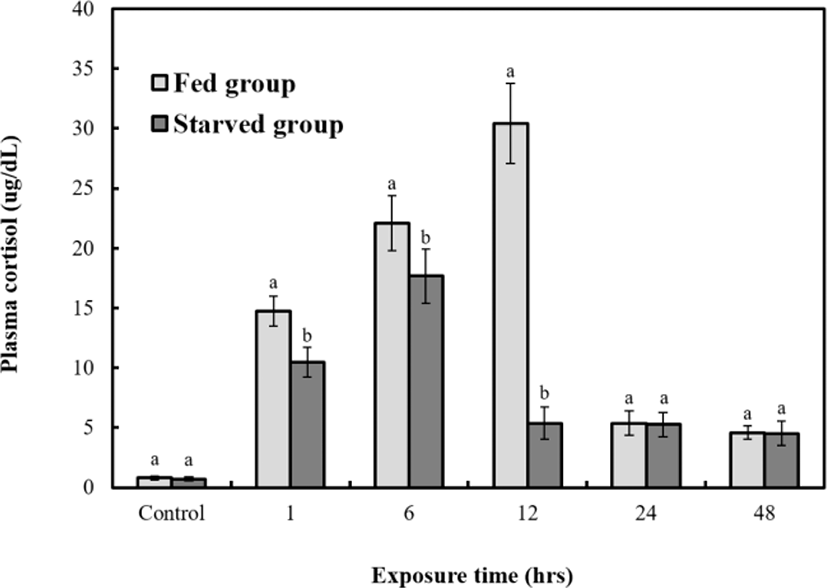
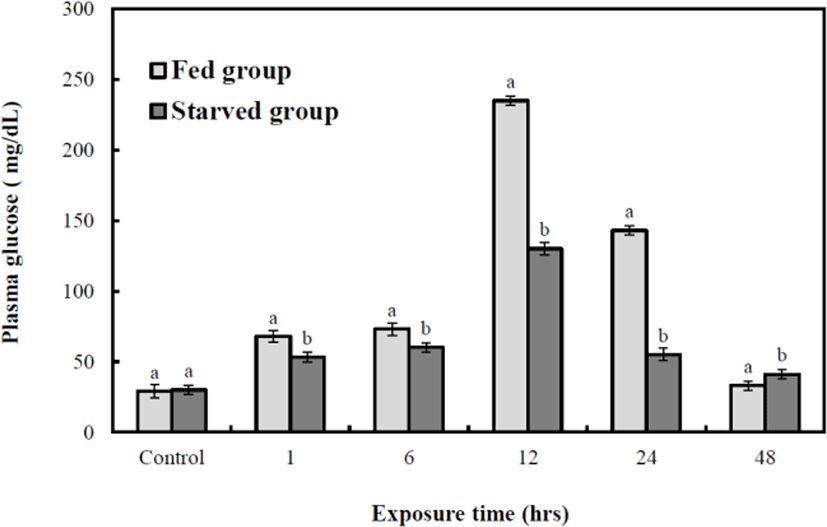
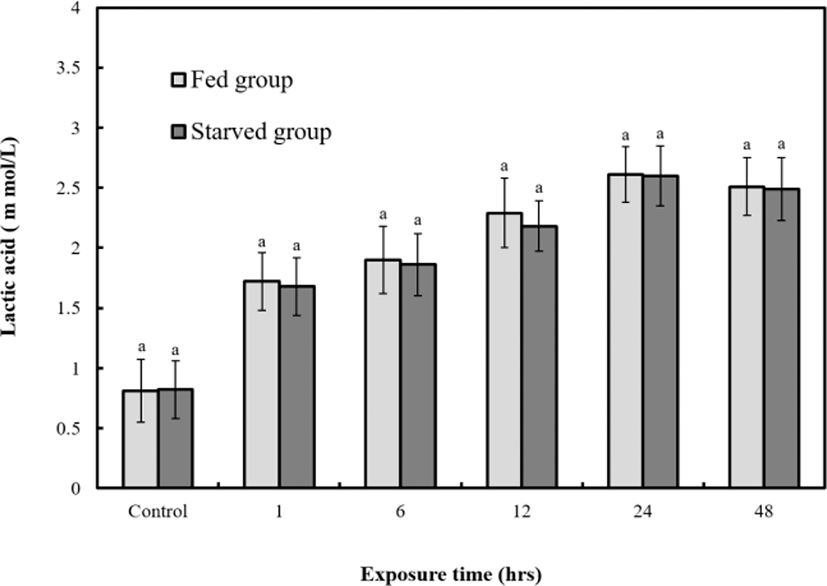
DISCUSSION
Larsson and Lewander (1973) noted that many fish undergo natural periods of starvation at a particular time every year and have consequently evolved the ability to withstand the prolonged food shortages. Such periods may amount to weeks, months, or even years, and may cause extensive loss of energy stores in the body as fish consumes its own tissues to remain alive (Weatherley & Gill, 1987; Park et al., 2015). This study shows that, Far Eastern catfish, Silurus asotus can tolerate the long term of food shortage for 210 days from the experimental proof.
When a fish is exposed to chronic stress, its metabolic reactions are altered by changes in the hypothalamic-pituitary-adrenocortical axis (HPA axis), including the hypothalamic secretion of corticotropin-releasing hormone (CRH) through the limbic system. CRH stimulates the release of adrenocorticotropic hormone (ACTH) from the pituitary gland, which then stimulates the release of cortisol from the target organ, and the interrenal gland which promots a metabolic stress reaction. Thus, cortisol is an important index of the stress reaction (Specker & Schreck, 1980). Severe starvation results in coma and catabolic disease of the gastrointestinal system. Basic metabolic reactions maintain energy level and body tissue (Jung et al., 2003). Guyton (1991) reported three stages of physiological change during starvation: in the first stage, the glycogen stored for urgent use is hydrolyzed, releasing glucose; in the second stage, acetyl-CoA is oversupplied, leading to acidosis; in the third stage, fish are compromised by protein exhaustion. In the process of starvation, sugar, lipid, protein, and other essential nutritional elements decrease rapidly, and finally, the whole abnormalities in immune, circulatory, and endocrine systems lead to death. In our study, dissolved oxygen concentration of starved groups decreased until 48 hrs, and fed groups until 16 hrs. Respiratory frequency also gradually decreased until 48 hrs. That is, respiratory function of all experimental groups decreased during the experimental period. Comparing the dissolved oxygen, pH, CO2, and NH4+ concentrations showed different trend, but rates of pH, CO2, and NH4+ concentrations change were similar to oxygen consumption rate. That is, the metabolic rate of all experimental groups decreased during experimental period.
The toxicity and effect of nitrite vary among fish species and depend on test conditions, such as fish size, water ion composition, and temperature (Doblander & Lackner, 1997). Chloride and other anions in water provide protective action against nitrite in active branchial uptake (Williams & Eddy, 1986); thus, small amounts of Cl–, for example, 1 mM, are likely to afford protection against high nitrite inputs (Eddy et al., 1983). The effect of sodium nitrite (NaNO2) on fish is more intense in Cl–-poor freshwater. Thus, NaNO2 is more toxic in freshwater than in seawater (Grosell & Jensen, 1999).
Plasma NaNO2 accumulation probably causes methemoglobinemia and malfunction of hemopoietic activity, which are detectable effects of NaNO2 intoxication (Costa et al., 2004). Although methemoglobinemia is not directly related to high mortality in fish exposed to NaNO2, the passing through of NaNO2 into the blood stream may cause an increase in blood cell lysis, changes in the plasma electrolyte balance, and efflux of K+ from red blood cells, which is evident from the increase in the number of shrunken RBCs (Knudsen & Jensen, 1997; Huertas et al., 2002; Martinez & Souza, 2002; Costa et al., 2004). The dysfunctional erythrocytes may be removed from the blood circulation because of an oxygen shortage, causing reduction in the total erythrocyte counts (Park et al., 2007). For this reason, a significant difference was caused between fed and starved group in this experiment.
Plasma cortisol and plasma glucose are recognized as useful indicators of stress in fish (Park et al., 2008). Plasma cortisol and glucose levels in red drum, Sciaenops ocellatus simultaneously exposed to stressor were reported to be elevated (Massee et al., 1995). Barton and Iwama (1991) stated that “In general, the phenomenon that plasma cortisol concentration of fish rises by stress is first order reaction, and the phenomenon that plasma glucose concentration rises is the second-order reaction by hormone rise caused by stress”. This finding has been reported in gray mullet, Mugil cephalus and kelp grouper, Epinephelus bruneus (Park et al., 2008). In this study, we have investigated the effect of starvation on Far Eastern catfish, exposed to the stress of NaNO2. In this study, it may safely be said that we have found out a useful index for recognizing the nutritional state of Far Eastern catfish in both fed and starved group experiencing the exposure to NaNO2.