INTRODUCTION
Aquaporins (AQPs) are a family of critical transmembrane proteins consisting of thirteen sub-types (AQP0-12) that are found in most living organisms including humans, animals, plants, and even lower organisms (Verkman, 2012; Shivaraj et al., 2017; Azad et al., 2021). In mammals, this protein family is ubiquitously expressed in various tissues and secretory glands specifically transferring water, glycerol, urea, or other soluble molecules across the cell membrane in a bidirectional manner to facilitate fluid absorption and secretion (Arnaoutova et al., 2008; Delporte, 2017). AQPs are playing an important role in the regulation of many cellular physiological processes including cell migration and proliferation (Meli et al., 2018), body water homeostasis (Yu et al., 2014), exocrine fluid secretion (Verkman, 2012), and transport the nutrients and other functional molecules into the cell, as well as elimination of metabolic residues (Ribeiro et al., 2021).
Many reports have demonstrated the expression of AQP proteins in the male reproductive system in different mammalian species such as humans (Pastor-Soler et al., 2001), rodents (Calamita et al., 2001a, 2001b; Lu et al., 2008; Ford et al., 2014), Bactrian camel (An & Wang 2016), stallion (Klein et al., 2013), bat (Oliveira et al., 2013), cat (Arrighi & Aralla 2014), and dog (Domeniconi et al., 2007). The expression and localization of AQP proteins in the male reproductive tissues are variable among the tested mammalian species (Boj et al., 2015; Carrageta et al., 2020; Ribeiro et al., 2022). However, it has been repeatedly addressed a potential role of AQPs in spermatozoa osmoregulation, transition, and concentration, as well as sperm maturation via regulating water homeostasis and fluid transport in the testes and male reproductive ductules (Lu et al., 2008; Alves et al., 2015; Carrageta et al., 2020). In support of this speculations, the deficiency or alterations in AQPs expression have been reported to be associated with sperm dysfunction and male sub-fertility. Chen et al. (2011) reported that AQP3 deficiency resulted in in utero sperm tail deformation and impairing male fertility in the AQP3-knockout mice. Also, the expression of AQP5 was found to be downregulated in the caput epididymidis from men with nonobstructive azoospermia compared to those from fertile men (Dube et al., 2008). Additionally, alteration of the AQP7 expression in infertile patients’sperm was found to be correlated with lower sperm motility, as well as abnormal sperm morphology and concentration (Saito et al., 2004; Yeung et al., 2010; Moretti et al., 2012). On the other hand, in a contradictory study, Sohara et al. (2007) reported that AQP7 knockout male mice were not sterile, and their sperm did not show any morphological or functional abnormalities. Moreover, although the knockout animals for some other AQP isoforms have been established and studied, their fertility has not been studied yet (Carrageta et al., 2020). Therefore, the role of these AQPs in sperm function and male fertility remains to be elucidated.
Although many efforts have been made to study the expression of AQPs in the male reproductive system using different assays, there is still a lack of the detailed expression location of these AQPs in the target cells along the male reproductive tissues (Carrageta et al., 2020; Ribeiro et al., 2022). Elucidating the detailed expression of the AQP proteins in the testes is a preliminary step to understand the functions of these AQPs in sperm production and maturation, and hence male fertility. Therefore, in order to extend the knowledge of the detailed expression and localization of AQPs, we investigated the expression pattern as well as the localization of different AQP subtypes in the adult mouse testes and testicular spermatozoa using an immunofluorescence assay.
MATERIALS AND METHODS
A total of 6 adult males of CD1 mice aged 10–16 weeks were used in the present study. The mice were kept at the animal resource center of Dong-A University Medical School with controlled lighting (12 h light/12 h dark) at a temperature of 20±2°C and humidity of 50±2%. The animals were provided with laboratory feed (SAM #31; Samtako, Osan, Korea) and water ad libitum. The protocols for this study were approved by Dong-A University Medical School Institutional Animal Care Use Committee (DIACUC-07-20).
The mice were euthanized by cervical dislocation. Then, the testes were exposed by laparotomy and meticulously isolated. After that, the tissue samples were embedded in Optimal Cutting Temperature medium (OCT) and rapidly frozen with liquid nitrogen. To prepare the sections, unfixed OCT-embedded frozen tissue blocks were sectioned at 5 μm thickness using a cryostat (Leica CM1950, Leica Biosystems, Nussloch, Germany). Hematoxylin and eosin staining following standard procedures was used for quality verification of the frozen sections. Then, the sections were mounted using VectaMount mounting medium (Vector Laboratories, Burlingame, CA, USA), and then observed using microscopy (BX51, Olympus, Tokyo, Japan). For further evaluation the slides were scanned with Panoramic MIDI digital slide scanner (3DHistech, Budapest, Hungary) and images were analyzed using CaseViewer.
The frozen sections were air-dried and fixed with ice-cold acetone for 30 min at −20°C. Then they were blocked with 1% bovine serum albumin in PBS containing 0.025% Tween 20 for 4 h at room temperature. The sections were incubated with the following primary antibodies overnight at 4°C; anti-AQP1 (1:200, Chemicon, Temecula, CA, USA), anti-AQP3 (1:200, Chemicon), anti-AQP7 (1:200, Novus Biologicals, Colorado, CO, USA), anti-AQP8 (1:300, Mybiosource, CA, USA), anti-AQP9 (1:200, Alpha Diagnostic Intl., San Antonio, TX, USA), and vimentin (1:200, Santa Cruz Biotechnology, Dallas, TX, USA) antibodies. After that, the sections were incubated with Alexa Fluor 488 or 594-conjugated anti-rabbit IgG H&L secondary antibodies (1:200, Invitrogen, Carlsbad, CA, USA) for 1 h at room temperature. Finally, the cell nuclei were counterstained with Hoechst 33342 trihydrochloride (1:500, Molecular Probes, Alameda, CA, USA). The sections were then mounted with prolong live antifade reagent (Invitrogen) and visualized using laser scanning confocal microscope (LSM 800, Carl Zeiss, Oberkochen, Germany).
RESULTS
The expression and localization of different AQP subtypes (AQP1, AQP3, AQP7, AQP8, and AQP9) in the adult mouse testes were explored using immunofluorescence assay. All the AQPs studied were expressed in the testes and revealed subtype-specific patterns in the expression intensity and localization depending on the cell types of the testes (Fig. 1). The overall expression intensity was highest in AQP7, followed by descending order of AQP3, AQP8, AQP9, and AQP1.
The expression intensity of AQP1 was the lowest in general among the tested AQPs. AQP1 was barely expressed in the germinal epithelium of seminiferous tubules, and weakly expressed in the interstitial Leydig cells. However, clear labeling of AQP1 was detected in the lumen of seminiferous tubules, corresponding to the tail and pericentriolar region of the testicular spermatozoa (Fig. 2).
The expression intensity of AQP3 was relatively high with broad distribution in the testis. Inside the seminiferous tubules, AQP3 expression was not with the same intensity. The strong AQP3 intensity was observed in the basal compartment of the tubules, corresponding to the spermatogonia and primary spermatocytes as well as Sertoli cells. Almost no signal was observed in the adluminal compartments. AQP3 expression was also observed in the lumen of the tubules, which coincides with tails of spermatozoa and elongated spermatids. Moreover, the expression of AQP3 was observed in the interstitial Leydig cells.
Intestingly, AQP7 was the most abundant AQP subtype among the tested AQPs that highly expressed in both intratubular and peritubular compartments of the seminiferous tubules. AQP7 was expressed in the all stages of germ cells of basal and adluminal compartments as well as in the Sertoli cells. Moreover, a strong AQP7 expression was also observed in the spermatids as well as in the head, pericentriolar region, and tail of the testicular spermatozoa.
AQP8 displayed a characteristic expression with localization to the basal compartment of the seminiferious tubules, corresponding to spermatogonia. AQP8 was also expressed in the interstitial Leydig cells with weak signals in the lumen.
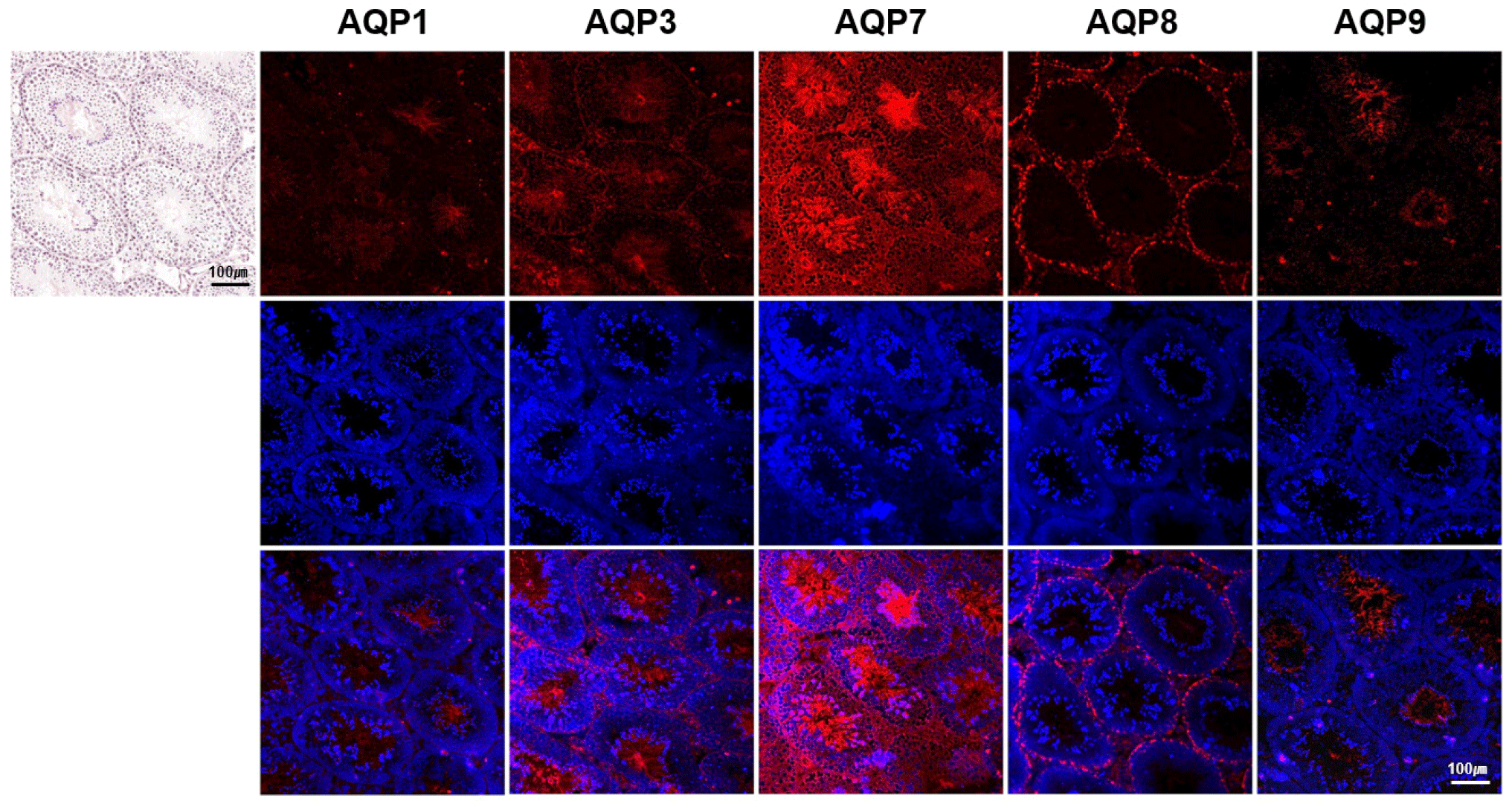
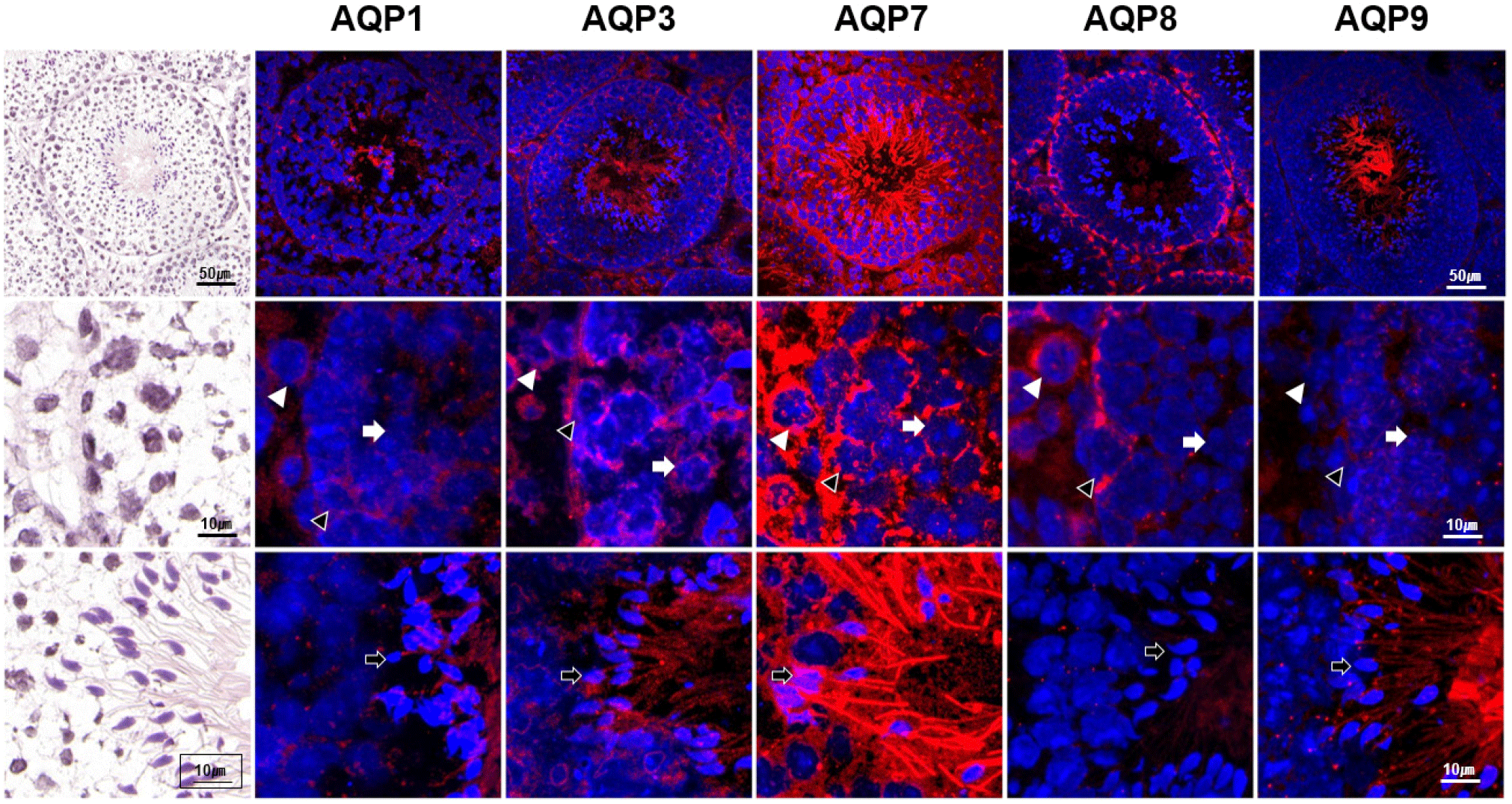
AQP9 expression was also distinctive, which was confined to the lumen of in the seminiferous tubules. Strong AQP9 expression was exclusively observed in tails of the testicular spermatozoa and elongated spermatids. Higher magnification revealed weak AQP9 expression in the Leydig cells.
In order to get more information on the detailed localization of AQP3, AQP7, and AQP8 expressions in interstitial and basal compartments of the seminiferous tubules, colcalization analysis of these AQPs was performed with vimentin, which is the most commonly used marker for Sertoli cells. AQP3 expression was identified in undifferentiated spermatogonia, proliferating primary spermatocytes, Sertoli cells as well as Leydig cells (Fig. 3). AQP7 was also expressed in the same cell types as AQP3, but the intensity of AQP7 was stronger than that of AQP3. AQP8 expression was limited to the spermatogonia and Leydig cells.
Relative expression levels of AQP subtypes in interstitial, basal and adluminal compartments of the seminiferous tubules were quantified in Fig. 4. Leydig cells in interstitial compartment expressed AQP7 at the highest level, followed by AQP8 and AQP3. Spermatogonia and primary spermatocytes in basal compartment expressed both AQP7 and AQP8 most abundantly, followed by AQP3. Spermatids and spermatozoa in adluminal compartment expressed AQP7 at the highest level, followed by AQP9 and AQP3.
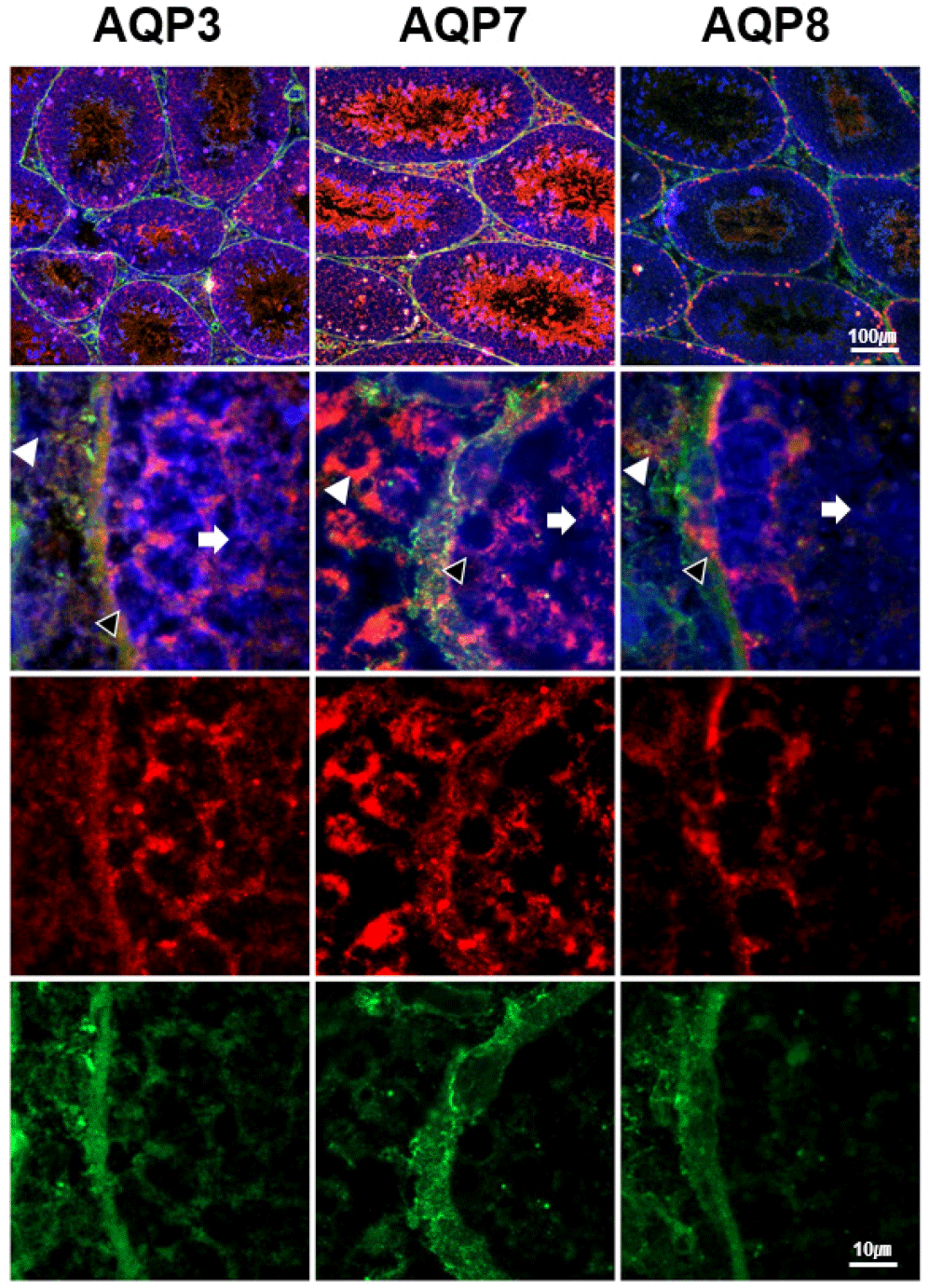
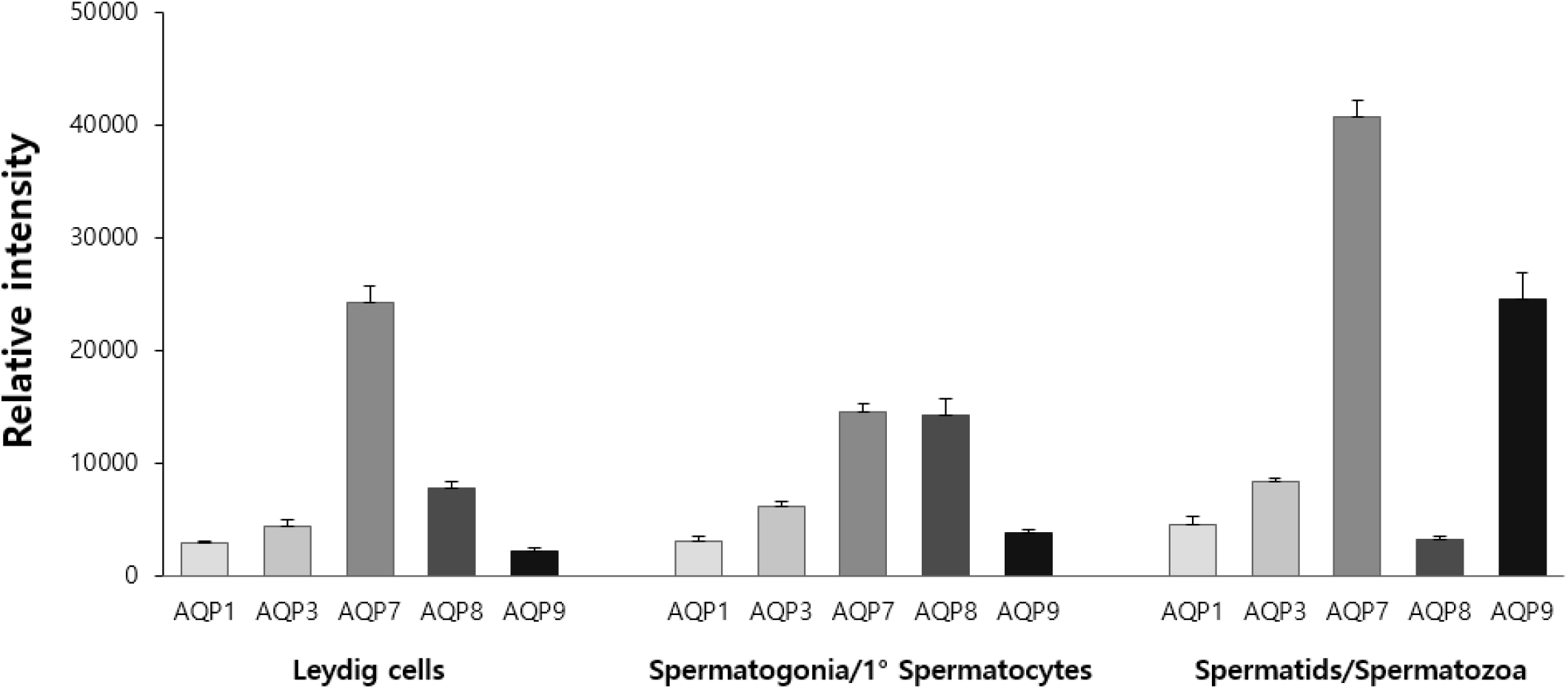
DISCUSSION
Investigating the AQP proteins expressed in the testes, and determining their localization in the target cells will help to predict and understand their specific roles in the central processes of fluid secretion and absorption which are mainly involved in spermatogenesis, sperm transition, and sperm maturation. Herein, our results provided a comprehensive description of the expression and localization patterns of different AQP subtypes in the adult mouse testes and testicular spermatozoa. It is noteworthy that the presence of AQP channels in the germ cells is involved, in somehow, in the rapid reduction in germ cells volume during spermatogenesis (Kageyama et al., 20001) due to the high osmolarity of the seminiferous tubules (Levine & Marsh 1971), and later participating in the sperm maturation and function (Chen et al., 2011; Squillacioti et al., 2021). AQPs may also be involved as one of the mechanisms facilitating the function of Leydig and Sertoli cells to regulate spermatogenesis via secretion and transport of the related hormones. Moreover, in Sertoli cells, AQPs may facilitate nutrients and fluid secretion to support germ cells nutrition as well as facilitate sperm transition through the lumen of seminiferous tubules (Nihei et al., 2001; Rato et al., 2010).
Among five AQP subtypes that were tested in the present study, AQP7 was the most abundant AQP protein expressed in the mouse testes. This finding was in accordance with those of Bernardino et al. (2018) who reported the intense expression of AQP7 at mRNA level in mouse testes using qPCR assay, and this expression was higher than those of AQP3 and AQP9. Herein, AQP7 was strongly expressed and observed in the germinal epithelium and Leydig cells, as well as overall the testicular spermatozoa. Surprisingly, these findings were not largely in accordance with those previously reported in humans (Yeung et al., 2009; Moretti et al., 2012; Laforenza et al., 2017), boar (Prieto-Marínez et al., 2016; Vicente-Carrillo et al., 2016), rat (Calamita et al., 2001a, 2001b; Hermo & Smith 2011), or even mouse (Skowronski et al., 2007); they reported the exclusive presence of AQP7 only in the round and/or elongated spermatid as well as in secondary spermatocytes in some rat strains (Kageyamaet al., 2001). Moreover, they also reported variable localization patterns of AQP7 in the sperm cells among the different species; the differences were also reported in the same species in the different studies. Notably, Calamita et al. (2001b) suggested that the possibility of a strain-to-strain and species-to-species variability should not be overlooked when comparing the AQPs expression.
Regarding AQP3, its expression in the mouse testes was not strong as AQP7; in spit of their localization was more similar. Like our findings, not strong expression of AQP3 was observed in the mouse testes at the mRNA level (Ma et al., 2000; Bernardino et al., 2018). Also, the localization of AQP3 in mouse testes in the present study was more similar to those observed in dog testes (Mirabella et al., 2021) and mouse sperm (Chen et al., 2011). Interestingly, although the expression of AQP7 in the mouse testes in this study was more abundant and stronger than those of AQP3 and according to the correlation between AQP7 expression and lower sperm motility as mentioned before, but we can suggest that AQP3 is more important for male reproduction, and AQP7 function during spermatogenesis is not indispensable and can be compensated by another AQPs or other different mechanisms. This suggestion is based on the findings that; the AQP7 knockout mouse was fertile with normal sperm concentration and morphology (Sohara et al., 2007). In contrast, Chen et al. (2011) reported the subfertility of AQP3 knockout mice and suggested that AQP3 is an essential membrane pathway optimizing postcopulatory sperm behavior.
Regarding AQP8, its expression was observed only in spermatogonium and Leydig cells. Surprisingly, several contradicted results have been published regarding the expression and localization of AQP8 in the rat testes and testicular sperm (Calamita et al., 2001a, 2001b; Elkjær et al., 2001; Tani et al., 2001; Badran & Hermo 2002; Yeung et al., 2009). However, to the best of our knowledge, this is the first report about the expression of AQP8 in Ledyig cells in mouse testes. Moreover, the expression of AQP9 in the present study was observed as a strong expression in tails of elongated spermatids and spermatozoa and weak expression in basal and adluminal and interstitial compartments of seminiferous tubules. Similar to our findings, AQP9 has been reported to be localized on boar sperm tail (Vicente-Carrillo et al., 2016), however, it has no specific staining pattern in human sperm (Yeung et al., 2009). Furthermore, except for the finding of Domeniconi et al. (2007) who reported that AQP9 is not expressed in the dog testes, many other authors reported the expression of AQP9 in the Leydig cells in humans (Arena et al., 2011), and rats (Elkjær et al., 2000; Nicchia et al., 2001; Badran & Hermo, 2002). According to these findings and ours, it seems that AQP9 is a common AQP in Leydig cells in humans and rodents. The presence of different AQP subtypes in the Leydig cells may suggest them as one of the mechanisms facilitating the endocrine function of Leydig cells to regulate spermatogenesis via secretion and transport the related hormones, as previously reported in the case of pituitary endocrine cells (Arnaoutova et al., 2008). Moreover, the presence of AQP8 and AQP9 on testicular spermatozoa may also suggest their involvement in sperm function. However, it seems that AQP8 is not indispensable for male fertility, because Yang et al. (2005) reported that the AQP8-knockout mice did not show impaired fertility or abnormalities in sperm count or morphology. Altogether, the abundant expression of the different AQPs in the male reproductive tissues and the fertility of AQPs knock-out mice may suggest that the AQPs are important proteins in the male reproduction, however the functional loss of one AQP subtype in the knock-out mice may be compensated by the other AQPs; especially when considering the fact that there are different AQP subtypes which have similar functions.
Finally, AQP1 expression in the testes was the weakest among the AQP subtypes tested. Lower expression of AQP1 was also previously observed in mouse testes at mRNA and protein levels using qPCR and Western blotting assays, but not using immunohistochemistry assay (Danyu et al., 2008; Lu et a., 2008). However, using immunofluorescence assay in the present study we could detect weak signals of the labeled antibody against AQP1 in the testes which may indicate to the higher sensitivity and specificity of the antibody used in the present study.
In summary, using the immunofluorescence assay in the present study allowed us to detect the high variation among the tested AQP protein subtypes regarding their expression and localization patterns in the mouse testes and testicular spermatozoa. Moreover, it is allowed to detect that some AQP subtypes are expressed and localized in the mouse testes and testicular spermatozoa with a specific patterns different than those previously reported in different mammalian species or even different mouse strains. Although some available data concerning some AQP subtypes were not consistent with our findings or even scarce. However, it seems that the AQPs expression and localization in the male testes and testicular spermatozoa is species/strain specific. On the other hand, the abundant expression of AQPs in the testes suggested that the AQPs are indispensable for male reproductive physiology. Meanwhile, these AQPs are working concurrently and the defect/loss of any AQP subtype may compensate for the other AQP subtypes. This suggestion is based on the fact that the AQP knockout mice are not sterile. Finally, further experiments investigating the detailed expression and localization of the different AQPs along the male reproductive organs in the different mammalian species are required to fully understand the role of these proteins in male fertility.