INTRODUCTION
As the number of coronavirus disease 2019 (COVID-19) vaccinations increases, various side effects such as fever, fatigue, headache, and arm pain have been reported. In particular, menstrual abnormalities were significantly higher among people who experienced other side effects related to COVID-19 vaccination (Muhaidat et al., 2022). Vaccination triggers an inflammatory response by an immune response, affecting the female reproductive system. Similarly, the COVID-19 vaccine also seems to affect women’s fertility by triggering an immune response. It is known that the COVID-19 virus itself can infect the ovaries, uterus, and vagina, disrupting women’s reproductive function and causing infertility and physiological disorders (Jing et al., 2020; Li et al., 2020). However, the overall side effects of the COVID-19 vaccine on the female reproductive system are still unclear.
Lipopolysaccharides (LPS) are large molecules consisting of a lipid and a polysaccharide that are bacterial toxins. The lipid portion of LPS is highly conserved in the middle and is recognized by membrane-bound TLR4 to induce cell reactions (Loppnow et al., 1989; Tobias et al., 1989; Raetz & Whitfield, 2002; Schumann, 2011). LPS acts as a prototypical endotoxin in monocytes, dendritic cells, macrophages, and B cells, promoting pro-inflammatory cytokines secretion (Mbongue et al., 2022). LPS causes a local and systemic inflammatory response. Injection of LPS increases the enumeration and differentiation of lymphocytes in the blood and the secretion of infectious cytokines (Singh & Jiang, 2003; Edey et al., 2016; Bulgari et al., 2017).
Over the years, many studies have been conducted on the effects of LPS on the female reproductive system. Induction of an immune response by administration of LPS in mammals has been reported to result in changes in ovarian function. LPS secrete inflammatory cytokines such as TNF-α, IL-1α, and IL-1β to stimulate apoptosis directly and inhibit steroid production, which has a detrimental effect on mammalian ovaries (Roby & Terranova, 1988; Gottschall et al., 1989; Pate, 1995; Kaipia et al., 1996). LPS stimulation induces fibrosis of the ovary and apoptosis of granulocytes (Lv et al., 2021). In addition, LPS affects the secretion of sex steroid hormones in both stromal cells and granulocytes, leading to ovarian dysfunction (Taylor & Terranova, 1996). The immune response from LPS administration negatively affects the uterus. Treatment with LPS induces local inflammation of the uterus, upregulates the TLR4 gene and protein expression, and promotes inflammatory cytokines (Menchetti et al., 2018). Administration of LPS causes DNA damage to the uterine cells and the preimplantation embryo, which can interfere with implantation by inducing inadequate preparation during the pre-pregnancy period in mice and humans (Jaiswal et al., 2009; Moustafa et al., 2019). LPS administration alters the proliferation of glandular epithelial cells, luminal epithelial cells, and stromal cells of the uterus, leading to implantation failure (Deb et al., 2005).
However, it still needs to be determined whether the COVID-19 vaccine has detrimental effects on women’s reproductive organs. Therefore, we investigated the effect of excessive immune response to a COVID-19 vaccine on reproductive function using a mouse model in which LPS was administered instead of the COVID-19 vaccine to induce a disproportionate immune response. As a result of LPS administration, ovulation was delayed, and apoptosis of ovarian and uterine cells increased. The expression of apoptosis-related genes and pro-inflammatory cytokine genes was upregulated in the ovaries and uterus of LPS-administered mice.
MATERIALS AND METHODS
Five-week-old female ICR mice were purchased from Koatech (Pyeongtaek, Korea) and housed under controlled illumination (12:12 h light/dark cycle, lights on/off: 8 h/20 h) and temperature (22±2°C). Mice were fed a standard rodent diet and tap water ad libitum. Animal care and experimental procedures were approved by the Institutional Animal Care and Use Committee at the Seoul Women’s University in accordance with guidelines established by the Korea Food and Drug Administration (SWU IACUC 2022-6).
Mice were administered with 5 IU pregnant mare serum gonadotropin (PMSG; Sigma-Aldrich, St. Louis, MO, USA) to synchronize their estrous cycles and a smear test was performed for one week to confirm that their estrous cycles were synchronized. Synchronized mice were selected and administered with LPS or saline 48 h after PMSG injection. As a control, mice were injected with saline 5 times at an interval of 1 day, and LPS-treated mice were injected with 10 μg LPS (Sigma-Aldrich) 5 times at an interval of 1 day. Mice were sacrificed 18 h after the last LPS injection, followed by ovaries and uterus obtained.
A smear test was performed to determine the estrous cycle of mice. The tail of the mouse was raised to reveal its vagina. Vaginal cells are flushed by gently introducing a small amount of PBS using a spoid, and a drop of flushed PBS was smeared on the slide. The estrous cycle was evaluated according to the shape of the vaginal cells observed under a light microscope.
The ovary and uterus were fixed in 4% paraformaldehyde, and then rinsed in ethanol series. After embedding in a paraffin block, the tissue blocks were cut into 10 μm sections. The tissue blocks were deparaffinized and rehydrated by sequentially treating xylene, and ethanol and then washed with PBS. Tissue slides were stained with Harris’ Hematoxylin (Muto Pure Chemicals, Tokyo, Japan) and Eosin Y solution (Sigma-Aldrich). All stained ovarian and uterine sections were observed under an optical microscope (YS100, Nikon, Tokyo, Japan).
Apoptosis in the ovary and uterus was assessed by TUNEL assay, which was performed by In Situ Cell Death Detection kit (Roche/Millipore-Sigma, Burlington, MA, USA). Paraffin sections of the ovary and uterus were deparaffinized, washed twice with PBS, and then stained with a TUNEL reaction mixture. After washing twice with PBS, the sections were counter-stained with DAPI. After washing twice with PBS, the stained sections were mounted with an anti-fade mounting solution (Vectashield, Burlingame, CA, USA) and observed under a fluorescence microscope (Zeiss Axioskop Upright, Oberkoche, Germany).
Samples of tissues were homogenized with RNA isoplus (TaKaRa Bio, Shiga, Japan). After chloroform extraction and isopropyl alcohol precipitation, RNA has dissolved in RNase-free DEPC (TaKaRa Bio) solution. The RNA concentrations were measured with the Nano-drop (Thermo Fisher Scientific, Waltham, MA, USA). First-strand cDNA synthesis was performed using the extracted RNA and oligo dT, followed by the double-strand synthesis in RT buffer (Invitrogen, Carlsbad, CA, USA) with dNTP (BIO BASIC, Markham, ON, Canada) and RTase (Invitrogen). qRT-PCR was performed in a buffer solution containing template cDNA, SYBR Green (Enzynomics, Daejeon, Korea), and each primer. Primer pairs (Bioneer, Korea) were as follows; 18S (Forward 5’-GTCTGTGATGCCCTTAGATG-3’, Reverse 5’-AGCTTATGACCCGCACTTAC-3’), Fas (Forward 5’-CTGCGATTCTCCTGGCTGTGAA-3’, Reverse 5’-CAACAACCATAG GCGATTTCTGG-3’), FasL (Forward 5’-TCCGTGAGTTCACCAACCAA-3’, Reverse 5’-TGAGTGGGGGTTCCCTGTTA-3’), IL-6 (Forward 5’-ACCAGAGGAAATTTTCAA TA-3’, Reverse 5’-TGATGCACTTGCAGAAAACA-3’), IL-1β (Forward 5’-GGTCAAAGG TTTGGAAGCAG-3’, Reverse 5’-TGTGAAATGCCACCTTTTGA-3’). The optimum temperature cycling protocol was determined to be 95°C for 10 s, 60°C for 10 s, and 72°C for 10 s using the Light Cycler 480 Real-time PCR System (Roche, Manheim, Germany).
RESULTS
The estrous cycle in mice was examined through a smear test. Each stage of the estrous cycle was determined according to the composition and morphology of vaginal epithelial cells and the number of erythrocytes. In the control mice, most of the estrous cycles were at the estrous phase, in which cornified epithelial cells and a small number of nucleated epithelial cells were observed (Figs. 1A and B). Most of the estrous cycles in the LPS-treated group were also in the estrous phase, and there was no difference between the two groups. As a result of observing the reproductive organs with the naked eye after opening the abdomen, both the control group and the LPS group showed swelling of the ovaries and uterus. In particular, the corpus luteum (CL) was observed in the control mice but not in the LPS-treated mice (Fig. 1C).
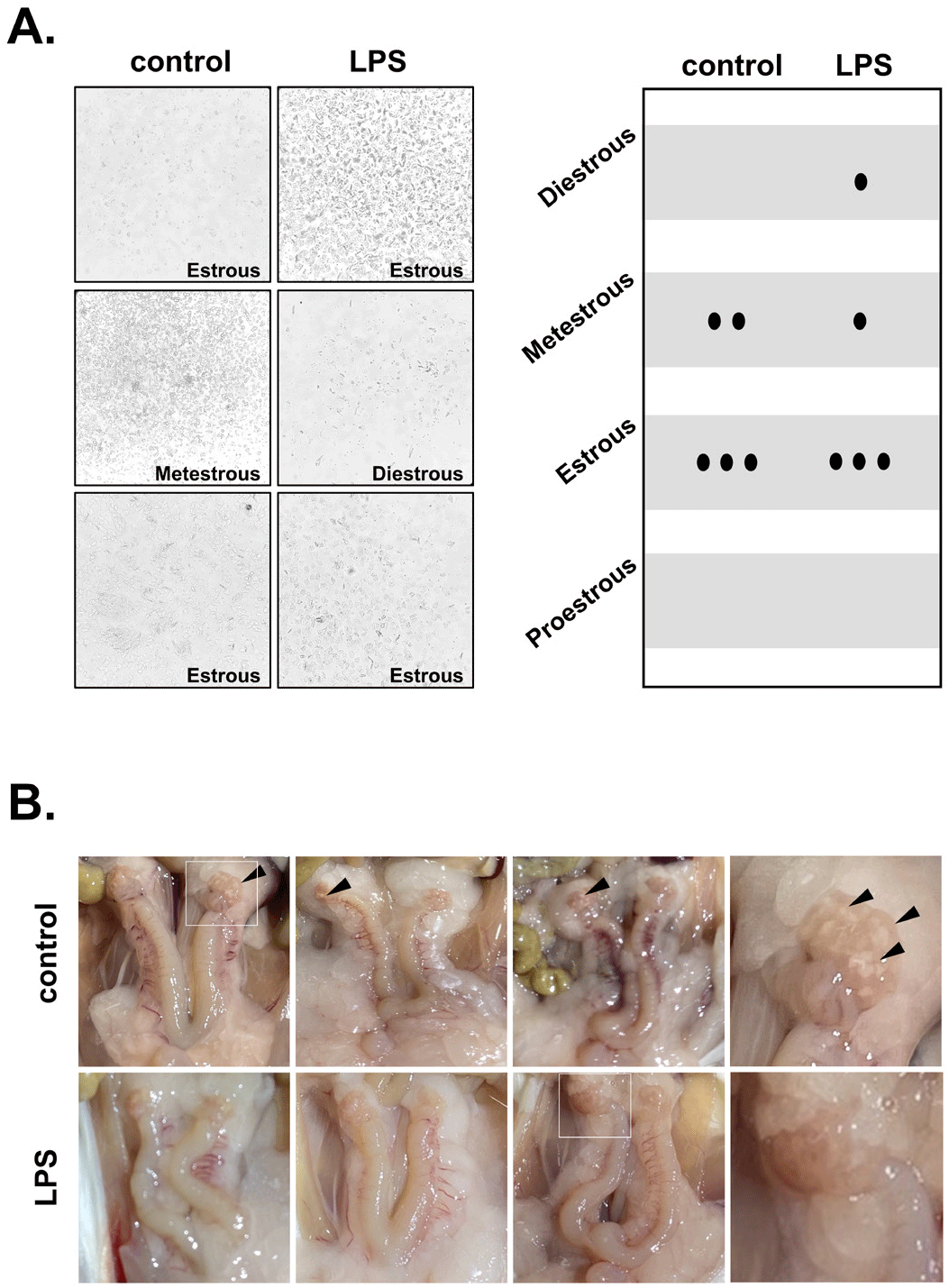
Ovaries and uterus were stained with hematoxylin and eosin, and then follicles were counted at each stage of follicle development to evaluate the effect of LPS on ovarian follicle development and uterine structure. In the ovaries of the control group, the proportion of primary follicles was 34.9%, secondary follicles 27.9%, antral follicles 11.6%, Graffian follicles 14.0%, and CL 11.6%. In the LPS-treated mice, the proportion of primary follicles was 44.0%, secondary follicles 28.0%, antral follicles 24.0%, Graffian follicles 4.0%, and CL 0%. The proportion of primary and antral follicles increased in the LPS-treated mice compared to the control mice. Conversely, the proportion of Graffian follicles was significantly decreased in the LPS-treated mice compared to the control. Moreover, no CL was observed in the LPS group (Fig. 2A). LPS administration also causes the endometrium and glandular epithelial cells to detach from the uterus and undergo cell death, thereby losing the shape of the uterine structure (Fig. 2B).
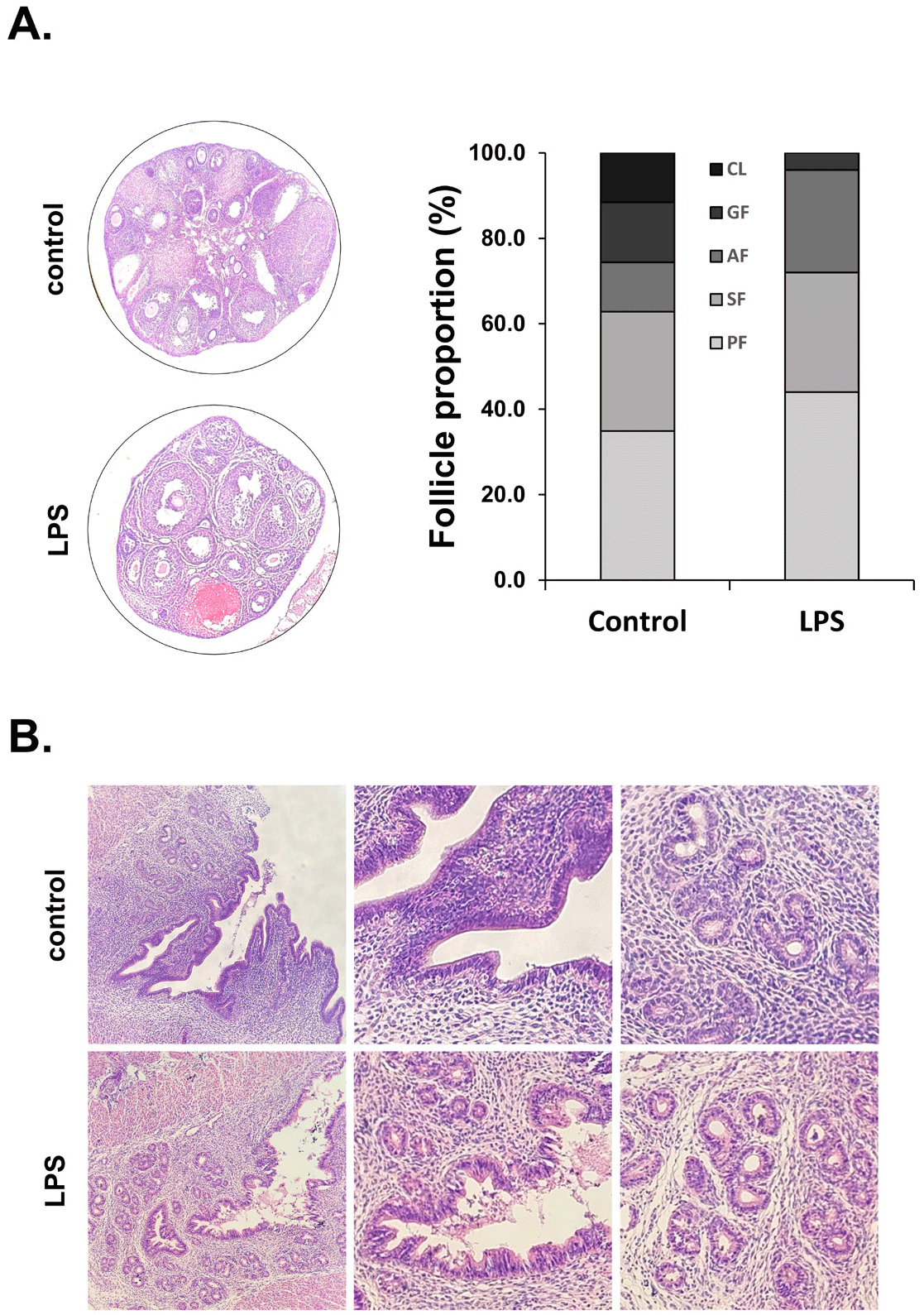
TUNEL assay was performed to evaluate uterine apoptosis. Many apoptotic cells were observed in the endometrium after LPS administration. In addition, many apoptotic cells were observed in the lumen of the uterus, in which the cells were epithelial cells detached from the endometrium (Fig. 3).
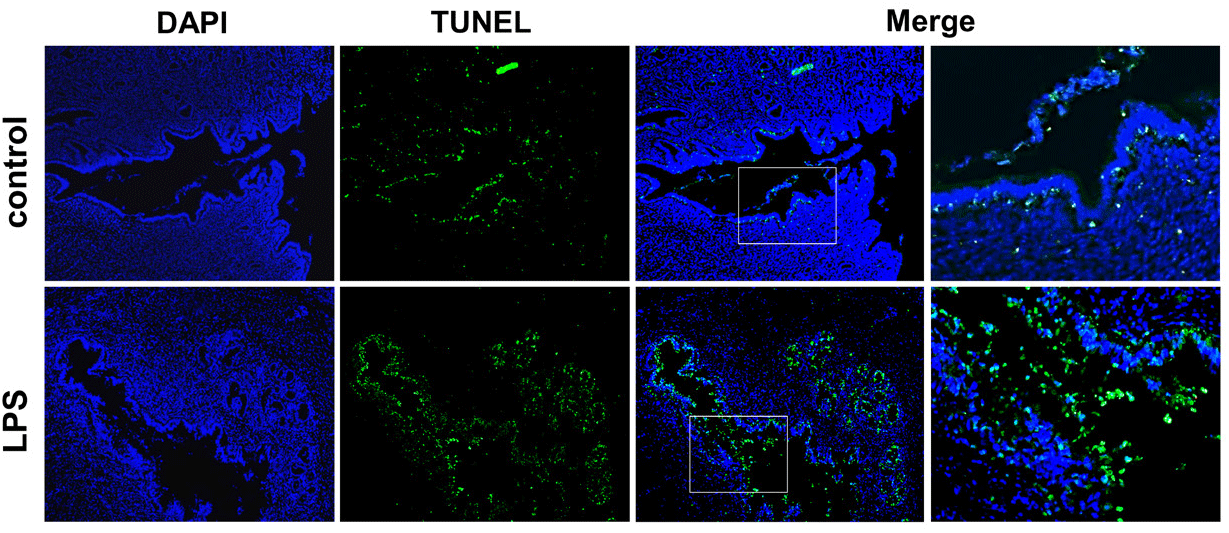
To elucidate the effect of LPS administration on the expression of apoptosis-related genes and pro-inflammatory cytokine genes in the ovary and uterus, Fas, FasL, IL-1β, and IL-6 mRNA were detected by qRT-PCR. The mRNA levels of Fas and FasL in the ovary were significantly higher in the LPS-treated mice compared to the control. The mRNA levels of pro-inflammation cytokines, IL-1β and IL-6, also increased in the ovary after LPS administration. Similar to the results of gene expression in the ovary, Fas, FasL, IL-1β, and IL-6 genes all increased in the uterus after LPS administration (Fig. 4).
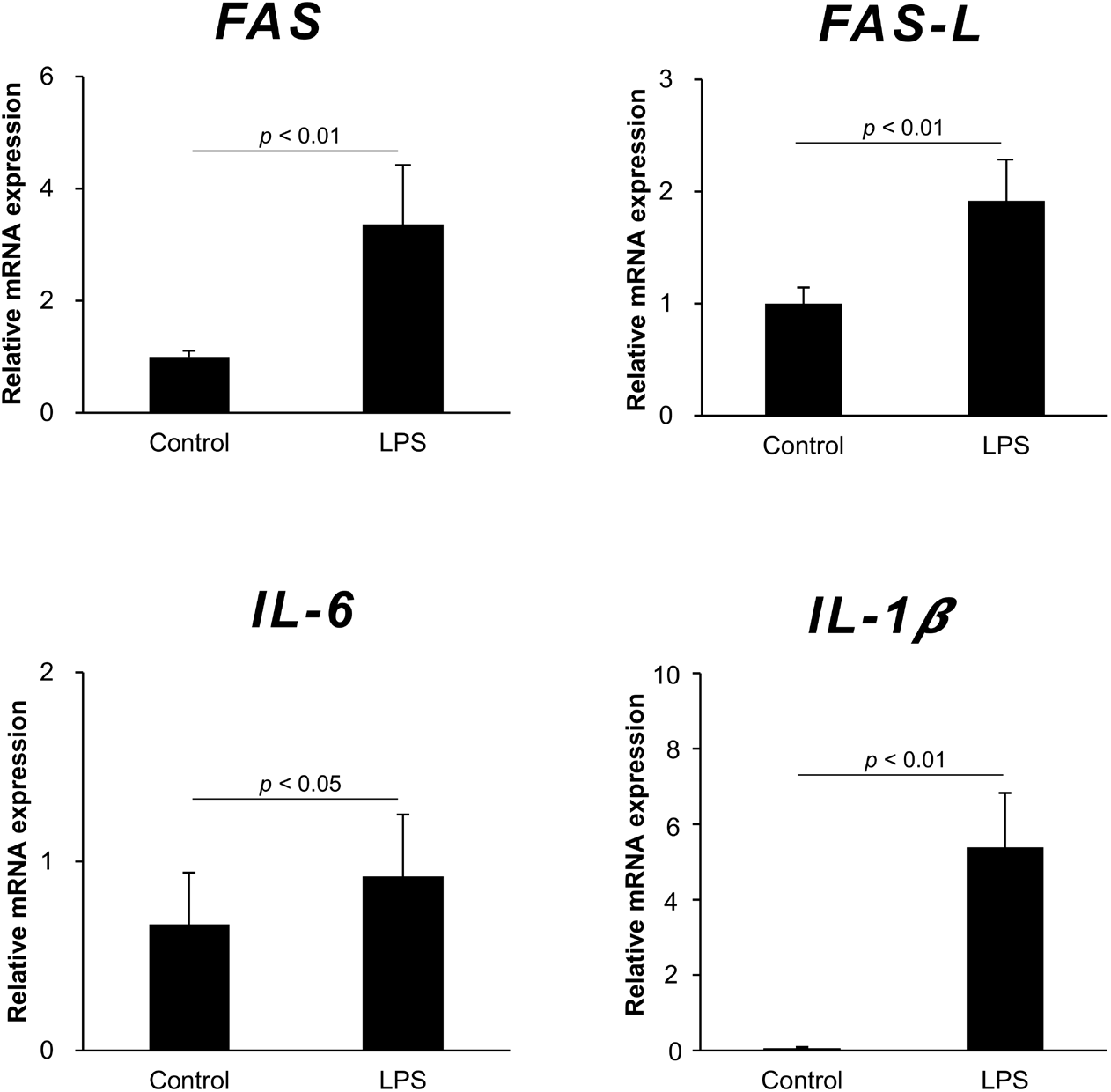
DISCUSSION
Many people are still getting the COVID-19 vaccine to prevent infection with the COVID-19 virus. Unfortunately, there are many reports of various side effects of the COVID-19 vaccination. One of these side effects is abnormalities in women’s reproductive function, such as irregular menstruation, amenorrhea, and resumption of menstruation (Muhaidat et al., 2022). However, the detrimental effects of the COVID-19 vaccine on women’s reproductive organs are not yet known. Therefore, we investigated the harmful effects of the COVID-19 vaccine on reproductive organs using an LPS-administrated mouse model.
As a result of histological observation of the uterus after administration of LPS to mice, the proportion of primary and antral follicles was increased in the LPS-treated mice compared to the control. In contrast, the number of Graffian follicles was significantly decreased in the LPS-treated mice. Interestingly, no CL was observed in the ovaries of LPS-treated mice. These results suggest that LPS administration may inhibit follicular development and ovulation. Consistent with our results, it has been reported that LPS administration to immature mice inhibits follicular development while delaying puberty (Yoo & Lee, 2006). In addition, LPS administration reduced LH secretion from the pituitary gland, which inhibited follicular development in the ovary (He et al., 2003). In rats, LPS administration caused to disrupt the estrous cycle by suppressing several steps in follicular development. Moreover, LPS is also known to slow the luteinizing process and delay ovulation (Suzuki et al., 2001). Our results showed that LPS administration inhibited follicular development and ovulation, but the estrous cycle did not differ between the LPS-treated mice and the control. Contrary to our results, there have been reports that LPS injection induces an excessive immune response, which may lead to an abnormal estrous cycle in cattle, ewes, and mice (Kalra et al., 1990; Peter et al., 1990; Battaglia et al., 2000). In our results, one of the reasons why there was no change in the estrous cycle may be due to the short duration of LPS administration. Another reason is that the estrous cycle was not investigated for a long time after the LPS administration.
On the other hand, when the uterus was observed after LPS administration, the endometrial cells were detached and the stromal cells were loose. As a result of apoptosis detection in the uterus by TUNEL staining, many apoptotic cells were detected in the endometrial epithelium after LPS administration. In addition, many apoptotic cells were observed in the lumen of the uterus. Similar to our results, it was reported that apoptosis increased and proliferation decreased during the decidualization process of the uterus of mice exposed to LPS. LPS significantly decreased the cell proliferation in the glandular epithelium, luminal epithelium, and stroma in the uterus during the preimplantation period (Deb et al., 2005). Moreover, it has been shown that LPS affects uterine function by binding to TLR4 expressed in endometrial cells (Sheldon & Roberts, 2010) and that LPS-induced TLR4 activation in the ovary inhibits E2 secretion, resulting in uterine collapse (Guan et al., 2021). On the other hand, LPS stimulates immune cells such as T cells and macrophages in mice to produce cytokines that affect the uterus and ovaries (Agostinis et al., 2019). LPS is also known to act as a potent regulator of the HPG axis at the pituitary level. LPS can not only directly affect LH synthesis, but also indirectly affect GnRHR expression, reducing pituitary responsiveness to GnRH stimulation (Herman et al., 2010). These results suggest that the uterus may be detrimentally affected by LPS directly or indirectly, leading to endometrial collapse and apoptosis of endometrial cells.
Next, we investigated the expression levels of Fas, FasL, IL-6, and IL-1β in the ovary and uterus after LPS administration. Fas is a cell-surface death receptor with an intracellular death domain that initiates apoptosis. The binding of FasL seems to cause trimerization of Fas and rapidly induces apoptosis (Nagata, 1998). Our result showed that Fas and Fas-L mRNA expression in the ovary and uterus were higher in the LPS-treated mice than in control mice. This result was consistent with the TUNEL assay result, supporting that LPS administration induces apoptosis in the uterus. IL-6 and IL-1β are (Turner et al., 2014). Meanwhile, the mRNA expression of IL-6 and IL-1β, key pro-inflammatory cytokines secreted from T cells, macrophages, and dendritic cells, was increased in the ovary and uterus after LPS administration. Similar to our results, it has been reported that intracervical treatment with LPS induces a moderate local inflammatory response in reproductive organ tissues (Menchetti et al., 2018). These results suggest that LPS may stimulate immune cells in the ovary and uterus to produce pro-inflammatory cytokines, which induces inflammation reactions in the reproductive organs.
The present study demonstrated that LPS had a detrimental effect on the uterus, increasing apoptosis and inflammatory response in the uterus and on the ovary, inhibiting follicular development and ovulation. These results suggest that LPS administration may cause disproportionate immune responses within the uterus and ovaries, resulting in an abnormal menstrual cycle, such as irregular menstruation, amenorrhea, and resumption of menstruation. Although LPS, an immune response inducer, was used as an alternative to the COVID-19 vaccine in this study, the results of this study provide evidence that excessive immune response caused by foreign substances such as the COVID-19 vaccine may induce reproductive function deterioration.