INTRODUCTION
The pharyngeal pouches are a transient epithelial structure essential for craniofacial development in vertebrates. They arise in the pharyngeal endoderm as a series of branches, which segment the pharyngeal arches and provide survival signals, such as sonic Hedgehog, to the cranial neural crest cells (cNCCs) in the arches. The segmented cNCCs then differentiate into the facial skeletal elements, including facial cartilages (Mork & Crump, 2015). The pouches, per se, further differentiate into endocrine glands in the head and neck, including the thymus and parathyroid (Grevellec & Tucker, 2010). Consistent with essential roles for the pouches in craniofacial development, defects in pouches led to severe loss or malformation of facial cartilages as well as hypoplasia of the thymus and parathyroid in mice and zebrafish (Piotrowski & Nusslein-Volhard, 2000; Dickmeis et al., 2001; Kikuchi et al., 2001; Grevellec & Tucker, 2010). In humans, abnormal pouches are an etiology of DiGeorge syndrome, a congenital craniofacial disability (Driscoll et al., 1992; Piotrowski & Nusslein-Volhard, 2000; Lindsay et al., 2001; Tran et al., 2011). Given the importance of pouches in craniofacial development, the genetic and cellular mechanisms controlling pouch formation are less understood in vertebrates. Genetically, Tbx1, Pax1, and Foxi1 transcription factors and Fgf, Wnt, Ephrin, and VEGF-C signaling molecules are essential for pouch formation (Lindsay et al., 2001; Nissen et al., 2003; Piotrowski et al., 2003; Crump et al., 2004; Ober et al., 2004; Tran et al., 2011; Choe & Crump, 2014; Okada et al., 2016; Jin et al., 2018; Liu et al., 2020). In zebrafish, Tbx1 initiates pouch formation by promoting a collective migration of pouch-forming cells through Wnt11r and Fgf8a (Choe et al., 2013; Choe & Crump, 2014). Migrating pouch-forming cells are then rearranged into a bi-layered structure by Wnt4 and Ephrin B2 and B3 ligands to mature pouches, with wnt4a expression being regulated by Foxi1 in zebrafish (Choe et al., 2013; Choe & Crump, 2015; Jin et al., 2018). In medaka and zebrafish, Pax1 regulates the expression of tbx1 and fgf3 in the pharyngeal endoderm to control pouch formation (Okada et al., 2016; Liu et al., 2020). Besides these transcription factors and signaling molecules, barely anything has been identified in pouch formation in vertebrates. Here, I tested the potential role of Neuropilins (Nrps) in developing pouches in zebrafish.
The Nrps are a family of cell surface receptors with many critical biological functions in the cardiovascular and neuronal systems (Guo & Vander Kooi, 2015). In vertebrates, two Npr family members, Nrp1 and Nrp2, are conserved and transduce VEGF and Semaphorin signals in the cardiovascular and neuronal systems (Guo & Vander Kooi, 2015). In angiogenesis, Nrps form a ligand/receptor complex, including VEGF and VEGFR (Guo & Vander Kooi, 2015). Nrp1 interacts with VEGF-A and VEGFR2 in mice and controls endothelial cell migration (Soker et al., 1998). In particular, it functions in endothelial tip cells as a downstream effector of a Notch signaling pathway (Fantin et al., 2013). The knockout of the Nrp1 gene in mice consistently led to embryonic lethality due to severe defects in endothelial cell migration (Jones et al., 2008). In zebrafish, knockdown of nrp1 has shown similar defects in angiogenesis (Lee et al., 2002). Nrp2 acts with VEGF-C and VEGFR2/3 in mice and is important for lymph angiogenesis (Yuan et al., 2002; Kärpänen et al., 2006). Npr2 functions in lymphatic tip cells and modulates tip cell extension at the cellular level (Caunt et al., 2008; Xu et al., 2010). Although a functional validation has remained, zebrafish Nrp2 also has been implicated in lymph angiogenesis as it is expressed in lymphatic vessels during embryogenesis (Yaniv et al., 2006). To transduce Nrp signals in endothelial cells, Mitogen-activated protein kinase (MAPK) has been implicated as a critical downstream effector of Nrp1 and Nrp2 (Wey et al., 2005; Bechara et al., 2008; Fung et al., 2016). Although the roles of Npr1 and Nrp2 are distinct, it has been implicated that they can compensate for each other in angiogenesis as the double knockout mouse displayed more severe defects in angiogenesis (Takashima et al., 2002). Besides VEGFs, Nrps are receptors for Semaphorin3 (Sema3) ligands. They act with the Plexin receptor to bind Sema3 and control axon guidance in the neuronal system (Parker et al., 2012). Sema3 ligands have been demonstrated as endogenous inhibitors of angiogenesis and lymphogenesis through Nrps (Mumblat et al., 2015; Nakayama et al., 2015; Yang et al., 2015). Indeed, Nrps integrate competitive VEGF and Sema3 signals to control endothelial cell migration (Serini et al., 2003; Tamagnone & Mazzone, 2011).
At the cellular level, the directed collective migration of endodermal cells initiates pouch formation, which is similar to the collective migration of endothelial cells in angiogenesis (Costa et al., 2016). Although VEGFRs have not been identified yet, the Vegf-C ligand is required for pouch formation in zebrafish (Ober et al., 2004). Furthermore, I found that Nrps and a Sema3 ligand were expressed in the pharyngeal endoderm during pouch formation in zebrafish. Based on this observation, I tested the potential role of Nrp signaling in pouch morphogenesis. Blocking the Nrp signal, specifically in the pharyngeal endoderm during pouch formation, led to severe defects in pouches and facial cartilages whose formation depended on appropriate pouch development. Knockdown of MAPK activities in the endoderm demonstrated defects in pouches and facial skeletons. This preliminary functional analysis suggests that Nrps are able to function in the pharyngeal endoderm to form pouches through MAPK.
MATERIALS AND METHODS
Zebrafish were handled according to the Animal Protection Act (2017) in Korea. All zebrafish work was approved by Gyeongsang National University Institutional Animal Care and Use Committee. Published lines include Tg(~3.4her5:EGFP) (Tallafuss & Bally-Cuif, 2003) and Tg(nkx2.3:Gal4VP16) (Choe et al., 2013). Tg(UAS:DN-Nrp1a), Tg(UAS:Sema3d), and Tg(UAS:DN-MAPK3) transgenic constructs were generated using the Gateway (Invitrogen, Carlsbad, CA, USA) Tol2kit (Kwan et al., 2007). For pME-DN-Nrp1a, a dominant-negative form of Nrp1a that is missing its cytoplasmic domain was produced by PCR using primers DN-Nrp1a-B1F2 and DN-Nrp1a-B2R from multi-stage zebrafish cDNA (Renzi et al., 1999). For pME-Sema3d, the coding sequence of sema3d was amplified using primers Sema3d-B1F2 and Sema3d-B2R from multi-stage zebrafish cDNA. For pME-DN-MAPK3, a dominant-negative form of MAPK3 was produced by fusion PCR with primers designed to produce a lysine to arginine substitution at lysine 85 (Izumi et al., 2001). 30 ng of transgenic constructs and 35 ng of DNA construct bearing Tol2 transposase gene were injected into one-cell stage wild-type Tübingen embryos. Three independent transgenic lines for Tg(UAS:DN-Nrp1a:pA), two for Tg(UAS:Sema3d:pA), and three Tg(UAS:DN-MAPK3:pA) were established based on α-crystallin:Cerulean eye fluorescence. Tg(UAS:DN-Nrp1a:pA)GNU125, Tg(UAS:Sema3d:pA)GNU141, and Tg(UAS:DN-MAPK3:pA)GNU152 were used for this study. Primers are listed in Table 1.
Immunohistochemistry for Zn8 (Zebrafish International Resource Center, 1:400), fluorescent in situ hybridization in conjunction with GFP immunohistochemistry (Torrey Pines Biolabs, Secaucus, NJ, USA; 1:1,000), and Alcian blue staining were carried out as described previously (Crump et al., 2004; Zuniga et al., 2011). Partial cDNA fragments of nrp1a, nrp2b, and sema3d were amplified from mixed-stage zebrafish cDNA. The PCR fragments were cloned into the pGEM®-T easy vector (Promega, Madison, WI, USA) and were confirmed by DNA sequencing. Digoxigenin (DIG)-labeled antisense riboprobes were transcribed with T7/SP6 RNA polymerase (Roche Life Sciences, Basel, Switzerland) from linearized plasmids. Primers used for riboprobes are listed in Table 2.
An Olympus FLUOVIEW FV3000 confocal microscope using FV31S-SW software (Olympus, Tokyo, Japan) captured fluorescent images for immunohistochemistry and in situ hybridization. Approximately 100 μm Z-stacks at 3.5-μm intervals were taken with an Olympus UPLXAPO 20X objective lens, then were projected into a single image. An Olympus BX50 upright microscope using mosaic V2.1 software (Tucsen Photonics, Fuzhou, China) imaged flat-mounted facial cartilages dissected manually from Alcian Blue staining larvae. Any adjustments were applied to all panels using Adobe Photoshop (Adobe Systems, San Jose, CA, USA).
Pouches and ceratobranchial (Cb) cartilages were scored to quantify defects in pouches and Cb cartilages. The normal pouch was scored as 1, abnormal and hypoplasic as 0.5, and missing as 0. Similarly, normal Cb was scored as 1, hypoplasic or fused Cb as 0.5, and missing Cb as 0. The multiple comparison test of Tukey–Kramer was employed to quantify defects.
RESULTS
There are four nrp genes, nrp1a, nrp1b, nrp2a, and nrp2b, in zebrafish. Previously, it has been reported that all four nrp genes are expressed in the brain at 24 to 72 hours-post-fertilization (hpf), with nrp1a and nrp2b being expressed specifically in mandibular cartilages at 36 to 72 hpf (Bovenkamp et al., 2004). However, the expression of nrp genes in the pharyngeal regions of the embryonic head has yet to be analyzed. To investigate a potential role of Nrp signaling in craniofacial development, I first examined the expression of nrp genes in the pharyngeal endoderm at 26 hpf, in which a series of pouches arises (Choe et al., 2013). To do so, I carried out in situ hybridization for nrp genes in wild-type embryos harboring Tg(her5:GFP) transgene, a reporter of the pharyngeal endoderm and pouches (Tallafuss & Bally-Cuif, 2003). When the posterior pouches formed at 26 hpf, nrp1a was expressed in the first pouch, with its expression not seen in other pouches (arrows in Fig. 1A). In addition, its expression was seen in the brain (arrowhead in Fig. 1A) and heart (asterisk in Fig. 1A). Transcripts for nrp2b were observed in the posterior pouches, including the second to fourth pouches at 26 hpf, whereas they were not seen in the first pouch (arrows in Fig. 1B). Besides, nrp2b was also expressed in the adjacent mesoderm to the pouches (arrowheads in Fig. 1B). Apparently, nrp1b and nrp2a were not expressed in the pharyngeal regions at 26 hpf. Next, I analyzed the expression of sema3 genes, ligands for Nrp receptors, in the endoderm during pouch formation. Among sema3 genes, I found that sema3d was expressed in the matured pouches as well as in the pharyngeal endoderm forming the fifth pouch at 26 hpf (arrows in Fig. 1C). Expression of nrp1a, nrp2b, and sema3d in the pharyngeal pouches at 26 hpf suggests that they could work together to form pouches during craniofacial development.
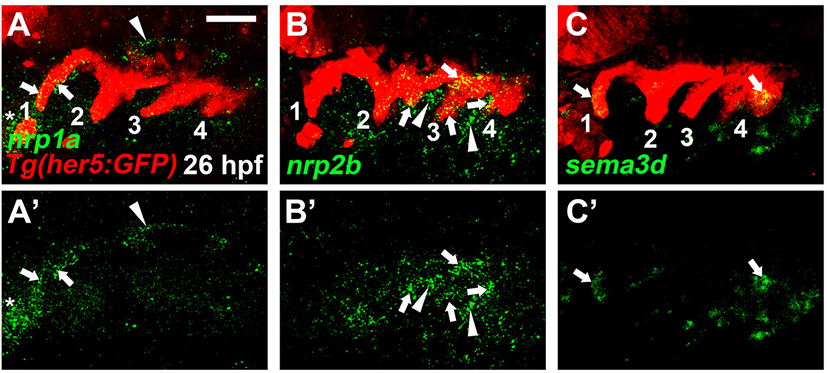
I utilized a dominant-negative approach to access the function of Nrp signaling in pouch formation. To do so, I generated Tg(UAS:DN-Nrp1a) lines harboring a dominant-negative mutant allele of nrp1a. Utilizing the pharyngeal endoderm driver Tg(nkx2.3:Gal4VP16) lines that are able to express target genes in pouch-forming endoderm, including the third and fourth pouches (Choe et al., 2013), I overexpressed DN-Nrp1a specifically in the endoderm during pouch formation. Overexpression of the DN-Nrp1a in the endoderm was expected for the endodermal cells to prevent them from receiving signals mediated by Nrps, including Nrp1a. In wild types, five pouches were visualized by Zn8 immunostaining at 34 hpf (Fig. 2A and D). However, compound animals bearing Tg(nkx2.3:Gal4VP16) and Tg(UAS:DN-Nrp1a) transgenes displayed abnormal pouches at 34 hpf (Fig. 2B). Although the first pouch was normal, the remaining pouches were hypoplasic, misshaped, or missing (Fig. 2B and D). In addition, pouches 3–5 were not separated from each other (arrows in Fig. 2B). This result suggests that Nrp signals are required in pharyngeal endoderm for pouch formation. I further verified the role of Nrp signals in pouch development by analyzing Cb cartilages whose formation relied on normal pouch development (Crump et al., 2004). Five pairs of Cbs formed symmetrically in wild types at 4 dpf (dots in Fig. 3A and D). In the compound animals, however, Cbs were missing or fused, confirming defects in pouches (arrows in Fig. 3B and D). Taken together with the expression of nrp1a and nrp2b in the endoderm and pouches during pouch formation, my functional analysis suggests a critical role of Nrp signals in developing pouches and facial cartilages.

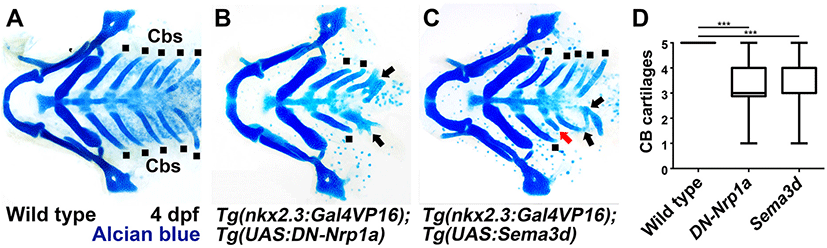
As I observed sema3d expression in developing pouches at 26 hpf, I also tested the potential role of Sema3d in pouch formation. To do so, I expressed sema3d ectopically in the pharyngeal endoderm during pouch formation by the UAS/Gal4 system. In compound transgenic animals harboring Tg(nkx2.3:Gal4VP16) and Tg(UAS:Sema3d) transgenes, pouches were missing or malformed compared to wild types (Fig. 2A, C, and D). For example, the first pouch was barely seen, and the fifth one was not completely separated from the fourth one (Fig. 2C). Consistent with defects in pouches, Cb cartilages were also fused to other Cbs or malformed in the compound animals (Fig. 3C and D). This result shows that the endodermal cells are able to respond to ectopically expressed sema3d during pouch formation, implying a potential role of Sema3d in pouch formation.
In the cellular contexts, activation of Nrp receptors promotes MAPK signaling, with Sema3 family ligands being required for MAPK activation (Wey et al., 2005; Bechara et al., 2008; Fung et al., 2016; Hai et al., 2022). Since I observed a potential requirement of the Nrp-Sema3d signal in pouch formation, I next examined whether MAPK was necessary in the endoderm for pouch development. To knock down MAPK activities in the endoderm during pouch formation, I overexpressed a dominant-negative form of MAPK3, specifically in the endoderm with the UAS/Gal4 system. Compared to wild types, compound Tg(nkx2.3:Gal4VP16) and Tg(UAS:DN-MAPK3) transgenic animals showed defects in pouches, including missing pouch and incompletely separated pouches (Fig. 4A, B, and E), and in Cbs such as fusion among Cbs (Fig. 4C–E). Interestingly, these defects were similar to those seen in transgenic animals knocking down Nrp signals in pharyngeal endoderm (Fig. 2B), implying a genetic interaction between Nrp and MAPK signals in the endoderm for pouch formation.
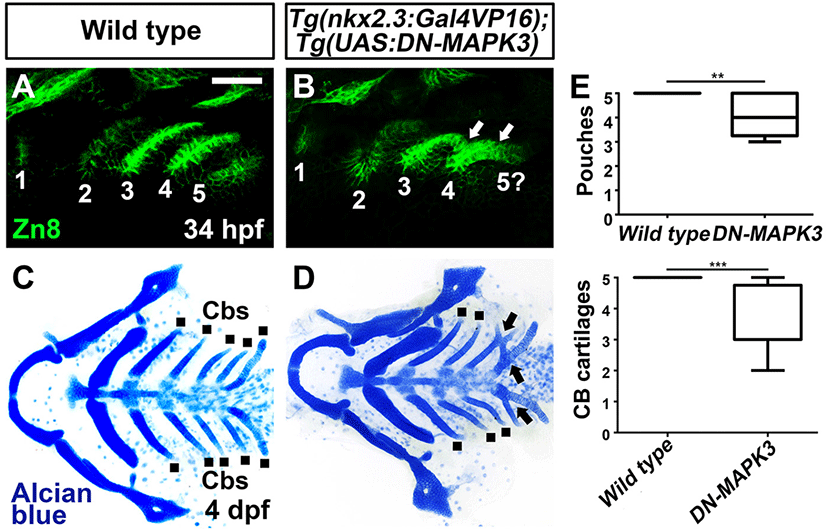
DISCUSSION
In this study, I examined a potential requirement of the Sema3d-Nrp-MAPK signal in pouch formation in zebrafish. Expression of nrp1a, nrp2b, and sema3d in pharyngeal endoderm, including pouches, suggests they could be necessary for pouch formation. Indeed, knockdown of Nrp signals or ectopic expression of Sema3d, specifically in the endoderm during pouch formation, resulted in defects in pouches and facial cartilages whose formation was dependent upon normal pouch formation. Furthermore, preventing MAPK activities in the endoderm showed similar defects in pouches and facial cartilages to those knocking down Nrp signals. Thus, it is feasible that the Sema3d-Nrp-MAPK signal is a critical signaling pathway controlling pouch formation in zebrafish. However, my transgenic analysis of the signal is unable to determine the role of the Sema3d-Nrp-MAPK signal in pouch formation for the following reasons. First, the dominant negative approach could knock down activities of Nrp family proteins simultaneously, but it cannot identify nrp genes necessary for pouch formation. Thus, to determine the role of Nrp signals in pouch formation, a loss-of-function (LOF) analysis of nrp1a and nrp2b genes using LOF mutants is required. Second, similar to the first reason, the Sema3d-Nrp signal cannot be determined in pouch formation without LOF analysis of sema3d. In particular, instead of Sema3d, Vegf-C that has been implicated in pouch formation could function in pouch formation through Nrps, as Vegf can transduce signals through heterodimer for VEGFR and Nrp (Soker et al., 1998; Ober et al., 2004; Guo & Vander Kooi, 2015). However, VEGFRs required for pouch formation have yet to be identified in zebrafish. Third, although MAPKs are essential downstream effectors to transduce Nrp signals, they are involved in transducing several signals from crucial growth factors, including EGF, TGF, and VEGF, in various cellular contexts (Zhang & Liu, 2002). Thus, analyzing genetic or molecular interactions between Nrps and MAPKs in pouch formation is necessary for determining the role of the Nrp-MAPK signal in pouch formation. Although it is still preliminary to identify a new signal to control pouch formation, my study suggests a possible role of the Sema3d-Nrp-MAPK signal in pouch formation. Currently, I am developing genetic and molecular tools to test the Sema3d-Nrp-MAPK signal in pouch formation at the genetic and molecular levels.