INTRODUCTION
As one of important female reproductive tracts, the uterus plays a role in variety of reproductive phenomena including embryo development, implantation, and pregnancy. They undergo physiological alterations including cytokines secretion, gene expression, angiogenesis, increase of number of secretory glands and thickness of endometrium during the estrous cycles (Stroband et al., 1986; Baker et al., 1998; Demir et al., 2010; Franczak et al., 2013). It secretes a various growth factors, cytokines, hormones, nutrients to regulate intrauterine microenvironment and these tissue remodeling in endometrium, which is induced by gonadotropins, steroid hormones, cytokines, and growth factors, provide a suitable environment in uterus for survival and development of embryo, and successful implantation.
Plasminogen activators (PAs) are one of serine proteases that convert inactivated plasminogen to plasmin and they are present as urokinase-type (uPA) and tissue-type (tPA) in extracellular fluids such as follicular fluid (Beers, 1975), uterine and oviductal fluid (Finlay et al., 1983; Kouba et al., 2000), and seminal fluid (Kobayashi et al., 1992). Activated plasmin degrades fibrin, fibronectin, and laminin, and activates other proteases such as collagenases and matrix metalloproteincase to degrade extracellular matrix (ECM). This feature of plasmin was involved in tissue remodeling (Martin & Arias, 1982), cell migration (Ploplis et al., 1998), activation of growth factors (Menshikov et al., 2006), and angiogenesis (Olofsson et al., 1998). Uterine and oviductal epithelial cells differently secreted two-type of PAs and their specific inhibitor (PAI) during the estrous cycle (Ahn et al., 2009; Hwangbo et al., 2013).
In female reproductive system, estrogen and progesterone play a crucial role in alteration of reproductive tract including uterus and oviduct for successful pregnancy. Estrogen is one of steroid hormones and it is transported from ovary to uterus through blood vessels. Moreover, it is binding to their specific receptors (estrogen receptors, ERs) in membrane or nuclear of inside cells (Elangovan et al., 2011), and this stimulation by estrogen is associated with initiation of estrous, development of reproductive organs, and promotion of uterine development for implantation (Gray et al., 2001). This hormone is existed as estrone, estradiol, and estriol in mammalians. Especially, 17β-estradiol (E2), secreted from porcine conceptus at pre-implantation period, regulates physiological conditions of uterine epithelial cells for successful implantation and it is important factor for maternal recognition in pigs (Bazer & Johnson, 2014).
Progesterone (P4) is steroid hormones secreted from corpus luteum. It is closely involved in estrous cycle, regulation of intrauterine environment for implantation and pregnancy, and maintenance of pregnancy (Spencer & Bazer, 2002). This hormone bind to their specific receptors (progesterone receptors, PRs), which are exist on membrane or inner nucleus, as well as estrogen and sequential action of these steroid hormones is a crucial role in luteolysis and alteration of physiological function in uterus (Spencer et al., 2004). During the pre-ovulatory phase, expression of PRs and ERs in uterine cells is increased by rapidly increased estrogen from ovary and increased concentration of P4 prevent ERs expression via binding with PRs (McCracken, 1980). These studies demonstrated that E2 and P4 are closely associated with regulation of functional and physiological conditions in endometrium as a key regulatory factors.
In human, mice, and cows, a number of studies regarding to express of PAs in uterus had been reported (Casslen & Astedt, 1983; Sappino et al., 1989; Tanikawa et al., 2009). However, regulation of PAs activity in porcine uterus is still unclear. Therefore, this study was conducted to investigate change of PAs activity and mRNA expression by P4 and E2 according presence of absence fetal bovine serum (FBS) condition in porcine uterine epithelial cells (pUECs).
MATERIALS AND METHODS
A porcine uterus at pre-ovulatory phase (Day 19–20 of estrous cycle) was collected from a local slaughterhouse and transported to the laboratory within 2 h on ice. The uterus was washed using Hanks’ balanced salt solution (HBSS) containing 0.06 g/L penicillin G and 0.1 g/L streptomycin sulfate, and it was separated from ovaries and mesometrium. Epithelial cells were isolated from luminal epithelium by gentle stripping with cell scraper and were incubated with Dulbecco's modified Eagle's medium/ nutrient mixture F-12 Ham (DMEM/F-12; Welgene, Gyeongsang-si, Gyeongsangbuk-do, republic of Korea) containing 66 units/mL Type IV collagenase (Worthington Biochemical Corporation, Lakewood, NJ, USA) at 38.5° for 30 min. To remove red blood cells, collected cells were mixed with Tris-NH4 lysis buffer (17.01 mM Trizma® base, 0.14 M NH4Cl) for 2 min at room temperature, then it were centrifuged at 1,500 g for 5 min. After centrifugation, the cells were washed by HBSS and cultured in phenol red-free DMEM/F-12 (Welgene) supplemented with 10 % (v/v) FBS (Welgene), 0.1 % (v/v) antibiotic-antimycotic (ABAM; Invitrogen, Carlsbad, CA, USA), 20 µg/mL amphotericin B (Sigma-Aldrich, St. Louis, MO, USA) in 100 mm culture dishes at 38.5° in a humidified atmosphere with 5 % CO2 until 80% confluence. Cultured pUECs were sub-cultured in 6-well culture plate at a density of 3×105 cells/well until 80% confluence for treatment. Then, cells were starved in serum-free DMEM/F-12 for 18 to 24 h and 20 ng/mL E2 (Sigma-Aldrich) and P4 (Sigma-Aldrich) with dimethyl sulfoxide (DMSO) in with serum or serum-free medium were treated to starved cells for 24 h. A supernatant and cells were collected and stored at −70° until analysis for PAs activity assay and quantitative real time RT-PCR, respectively.
Extraction of RNA from pUECs was carried out using trizol reagent (Takara, Shiga, Kusatsu, Japan) and cDNA was synthesized by PrimeScriptTM 1st strand cDNA Synthesis Kit (Takara) according to manufacturer's instructions. In brief, 1 µg of total RNA were incubated with 5 µM Oligo dT primer and 1 mM dNTP mixture at 65° for 5min, and it was immediately cooled on ice after incubation. Then, RNA was mixed with 1 units/µL RNase inhibitor and 10 unit/µL RTase, and mixture was reacted to synthesize cDNA at 42° for 1 h. Synthesized cDNA was quantified using NanoDrop 2000 (Thermo Scientific Nanodrop, Wilmington, DE, USA) and was diluted concentration at 50 ng/µL for real time PCR.
All of primers used in this study were designed by Primer3 online software (Table 1). Thunderbird SYBR qPCR mix (Toyobo, Osaka, Japan) was used to real time RT-PCR and cDNA amplification was performed following three steps: denaturation at 95° for 15 sec, annealing at 60° for 30 sec, extension at 72° for 30 sec, 40 cycles. Relative expression levels of urokinase-type PA (uPA), tissue-type PA (tPA), uPA specific receptor (uPAR), and type-1 PA inhibitor (PAI-1) mRNA were normalized to glyceraldehyde-3-phosphate dehydrogenase (GAPDH) and analyzed by 2−ΔΔCt method.
Samples of the collected culture medium (20 µL) were dispensed into a 96-well microplate and mixed with 30 µL of a plasminogen working solution (2.5 µg/well plasminogen; Sigma-Aldrich). The solution was incubated at 38° for 1 h. After incubation, 200 µL of substrate buffer [0.18 mM Z-L-Lys-SBzl hydrochloride, 0.22 mM 5,5′-dithiobis-(2-nitrobenzoic acid), and 0.01% Triton X-100] was added and was subsequently incubated at 38° for 1 h. PAs activity was determined by absorbance at the wavelength of 405 nm using a microplate reader.
-
Experiment 1: Alteration of effect of E2 and P4 on PAs activity according to presence or absence of FBS in culture medium
To compare effect of steroids on PAs expression and activation by FBS, pUECs, which were cultured at 50% confluence, were starved for 18 to 24 h. Then, they were incubated in culture medium with or without FBS containing 20 ng/mL E2 or P4 for 24 h. In experiment 1, both of steroid hormones were diluted with DMSO and final concentration was at 0.1% (v/v) DMSO in treatment groups. Because FBS contain several protease inhibitors and plasmin is one of proteases, we compared the pattern of PAs activity and expression with or without FBS. As a control groups, we prepared serum control and serum-free control without DMSO.
-
Experiment 2: Effect of E2 and P4 on PAs activity in culture medium with reduced concentration of DMSO
Based on results in experiment 1, we expected that 0.1% DMSO weakly influenced to expression of PAs-related genes. To identify more precise effect of steroid on PAs activity in pUECs, steroid hormones were diluted with reduced concentration of DMSO (0.002%; v/v). In experiment 1, we confirmed that pUECs sensitively response to steroid hormones in FBS absence condition. Thus, only serum-free medium was used in experiment 2. Concentration and treatment time of hormones were followed to experiment 1.
All numerical data representing each parameter were analyzed using Statistical Analysis System software (SAS, version 9.4). Data are represented as the means±standard error of the mean (SEM). Data from experiment 1 were analyzed using Duncan's multiple range test, and t-test was used for experiment 2. Comparisons among treatment groups were conducted using a generalized linear model in the SAS package. A value of p<0.05 was considered statistically significant.
RESULTS
-
Action of hormones on PAs activity by presence or absence of FBS in culture medium
The effect of sex steroids, which were diluted with 0.1% DMSO, in different serum condition on PAs-related genes expression and activation of PAs shown in Figs. 1 and 2. The expression of PAs-related genes were not changed by hormones in both of serum treatment groups. Treatment of E2 in serum-free group increased PAs activity compared to 0.1% DMSO treatment (p<0.05), whereas it was not altered in serum group.
-
Effect of E2 and P4 on PAs activity in culture medium with reduced concentration of DMSO
Change of PAs mRNA expression and activity by steroid hormones with reduced DMSO concentration shown in Figs. 3 and 4, respectively. Only expression of tPA mRNA was increased by E2 treatment (p<0.05) and 0.002% DMSO did not affect to mRNA expression. And treatment of E2 enhanced PAs activity compared to control group that was without DMSO treatment (p<0.05).
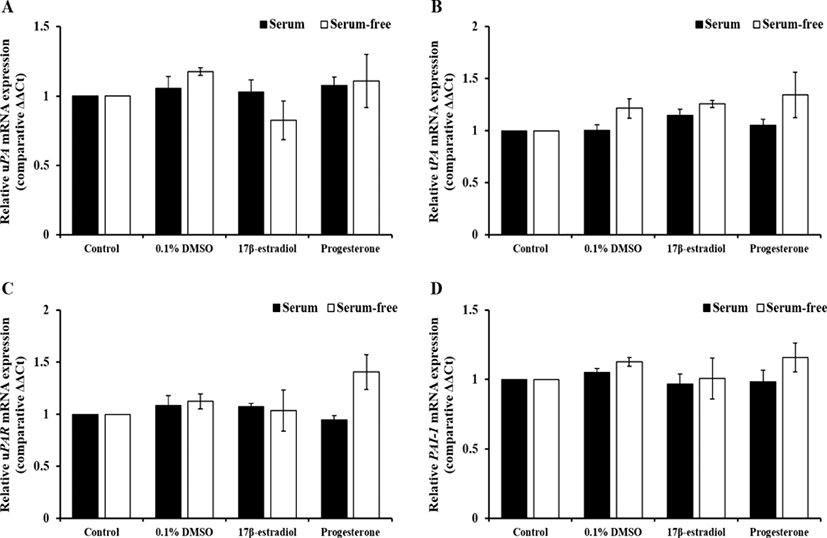
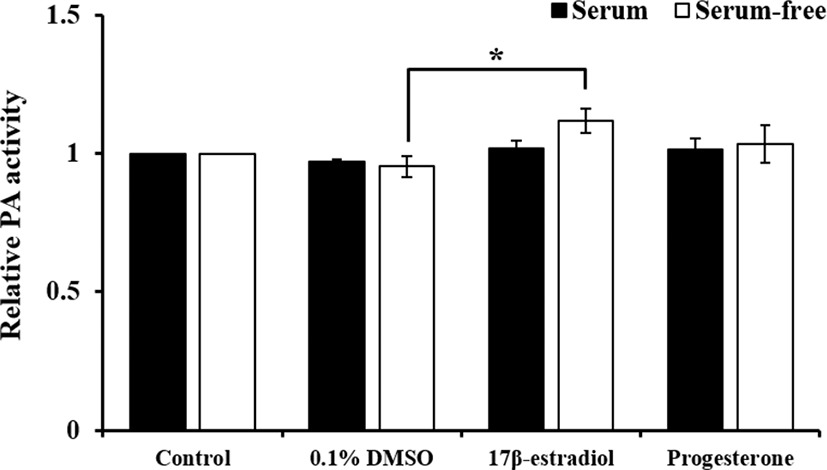
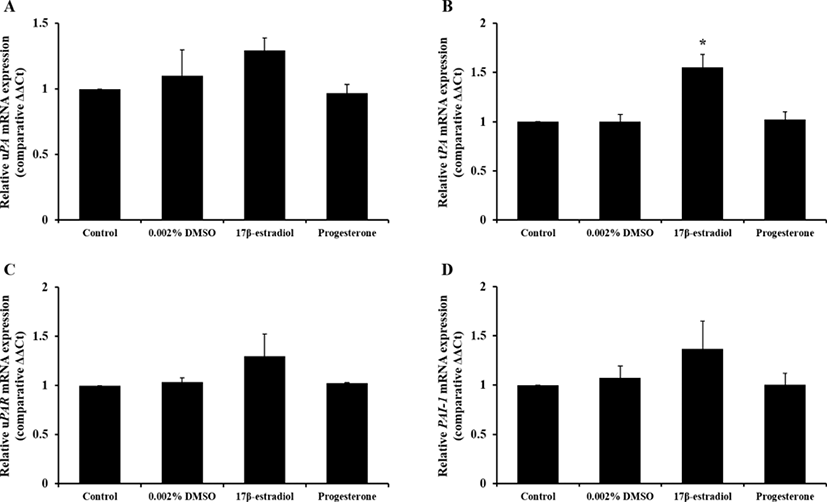
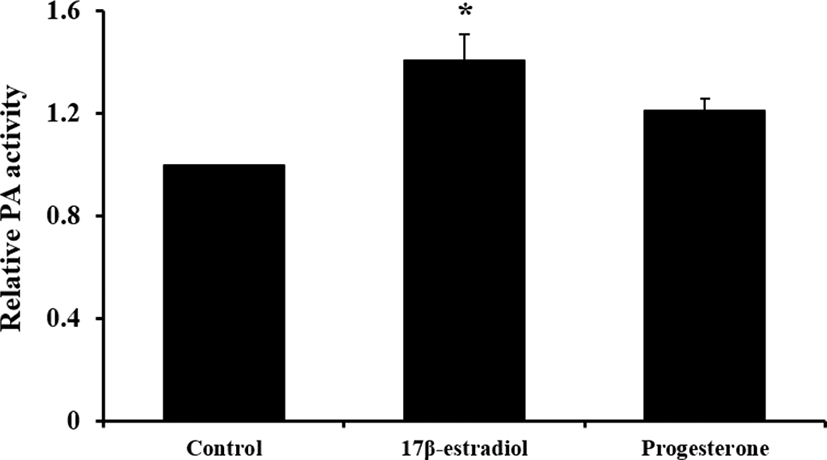
DISCUSSION
In mammalians, physiological and morphological features during estrous cycle were altered by various chemokines, cytokines, growth factors, and hormones for successful pregnancy. Cheon (2007) reported that collagen in murine uterus was regulated to induce suitable environment for implantation during early pregnancy. As one of tissue remodeling factors, PAs were existed in porcine uterus and expression of PAs was changed during estrous cycle (Kim et al., 2011). It was involved in reproductive and physiological phenomena including degradation of ECM proteins, angiogenesis, ovulation, and oocyte maturation (Ebisch et al., 2008). Krania et al. (2015) reported that supplementation of tPA during in vitro fertilization of bovine oocytes decreased embryonic development and increased expression of apoptosis-related genes. These demonstrated that PAs are closely associated with reproductive phenomena and play an important role for successful embryo development, fertilization, and pregnancy. Therefore, understanding of expression and regulatory mechanism of PAs in female reproductive tract and cells is crucial.
This study was conducted to confirm effect of steroid hormones on activation of PAs and PAs-related genes expression in pUECs at pre-ovulatory phase. Both of steroid hormones that were diluted with 0.1% DMSO did not affect to mRNA expression of PAs-related genes regardless of addition of FBS, however, action of hormones was stronger in serum-free condition. The treatment of E2, diluted with 0.002% DMSO, in serum-free medium increased tPA mRNA expression and PAs activity.
Sex steroids including estrogen, P4, and androgen play a crucial roles in function and growth of endometrium (Cheon et al., 2009). During endometrial growth in mice, treatment of estrogen increased the thickness of luminal epithelium, indicating estrogen acted as one of important factors for development of female reproductive tracts (Lai et al., 2000). In addition, action of estrogen in uterus influenced secretion and expression of growth factors, cytokines, and other molecules as well as endometrial development. Estrogen in porcine endometrium regulated the expression of keratinocyte growth factor, ERs, vascular endothelial growth factor (VEGF), and relaxin receptor for successful pregnancy (Ka et al., 2001; Yan et al., 2008). White et al. (2005) had report that osteopontin, important factor for attachment between porcine conceptus and endometrium, in luminal epithelial cells was enhanced by estrogen treatment in pregnant sows and it demonstrated that estrogen derived from porcine conceptus could induce expression of osteopontin in endometrium for successful pregnancy.
P4 is an important sex steroid for maintenance of pregnancy, and it regulate the cellular function and gene expression in variety of cells via interaction with their receptors as well as estrogen (Bazer et al., 2008). The expression of fibroblast growth factor 10 (FGF10) was stimulated by P4 in uterine stromal cells. Secreted FGF from stromal cells induced secretory response and gene expression through activation of phosphoinositide 3-kinase (PI3K) and mitogen activated protein kinase (MAPK) signaling pathway in uterine epithelial cells, and it was involved in cell migration, proliferation, and differentiation of trophoblast cells (Bazer et al., 2008). As mentioned above, action of P4 decreased expression of ERs (McCracken et al., 1980).
In present study, PAs activity did not influenced by P4, whereas E2 stimulated activation of PAs. In particular, expression of tPA mRNA was increased by E2 treatment. In porcine oviductal and uterine tissue, PAs activity was higher in pre-ovulatory phase than luteal phase (Ahn et al., 2009; Kim et al., 2011) and these findings suggested that E2 and follicle-stimulating hormone could enhance PAs activity in luminal epithelial cells through increase tPA expression and increased activity might be involved in endometrium development, growth of glandular epithelium, and angiogenesis during estrous cycles in pigs.
Mullins et al. (1980) and Fazleabas et al. (1982) reported that expression, secretion and activation of PAI in porcine uterus were induced by P4. Similar with porcine uterus, administration of P4 increased PAI-1 expression in porcine oviduct tissues (Kouba et al., 2000). However, in this study, P4 did not affect to PAs activation in epithelial cell, whereas E2 stimulated PAs activity. In human, activation of PAs in uterus was regulated by PAI-1 during decidualization of stromal cells, and PAI-1 expression in cultured stromal and decidual cells was increased by progestin (Schatz & Lockwood, 1993; Schatz et al., 1995). The PRs levels in epithelial cells in ovine uterus was lower than stromal cells and it was increased by stimulation of estrogen (Bazer et al., 2008). Spencer et al. (1995) reported that expression of PRs was reduced by continuous exposure by P4. In pig, physiological concentration of P4 in blood plasma is approximately 1 ng/mL at pre-ovulatory phase (Noguchi et al., 2010). Therefore, we supposed that P4 did not affect to express and activate of PAs in epithelial cells, because absence of stromal cell and estrogen lead to reduce expression of PRs in epithelial cells and these were caused decreasing of response by P4 stimulation. And high concentration of P4 might decreased PRs level in cells via negative feedback. To investigate effect of P4 on regulation of PAs activity, our futher plan include analysis of expressino of PRs under co-incubation of epithelial and stromal cells, and concentration and treatment time.
The FBS is commonly used to supply nutrients and support survival, divide, and growth of various type of cells. It contains a variety of proteins, growth factors, and several undefined factors. In our findings, PAs activity was not affect by both of E2 and P4 under FBS-presence condition. Alpha-2-macroglobulin (α2M), one of components in FBS, inhibits several proteases such as plasmin and kallikrein (de Boer et al., 1993). Therefore, because of several protease inhibitors including α2M in FBS, changes of PAs activity by both steroid hormones was not detected. However, more researches are needed whether FBS inhibits function of steroids or action of proteases such as plasmin.
In conclusion, we found that effect of steroid hormones is strongly in serum-free condition than serum condition and PAs activity was enhanced by estrogen, whereas P4 did not affect. The Serum-free condition was proper to measure activity of protease by steroid hormones because various components in FBS could influence to express receptors for hormones and activation of protease. The activation of PAs was increased by treatment of E2 via increasing expression of tPA and it may be contribute to uterine development during pre-ovulatory phase in pigs.