INTRODUCTION
The importance of the role of estrogens in the sexual development and reproduction of vertebrates is well known, and in particular, estrogens broadly contribute to regulation of female reproductive system, secondary sex characteristics during puberty, and ovulation (Rasier et al., 2006). Biosynthesis of estrogens appears to occur via the cytochrome P450 aromatase enzyme complex in diverse vertebrates, and it is generally accepted that estrogens are effectors for the reproduction strategy, such as implantation, pregnancy recognition, pregnancy maintenance, parturition, and lactation (Lange et al., 2002). Estradiol-17β (E2) is an estrogen and the major female sex hormone involved in the regulation of the estrous and menstrual female reproductive cycles. The biological effects of E2 occur through binding with estrogen receptors (Ers; Evans, 1988).
ERs belong to the superfamily of nuclear receptors and specifically to the family of steroid hormone receptors that act as ligand-mediated transcription factors (Evans, 1988; Zhao et al., 2008). Two forms of the ER, ERα, and ERβ, have been characterized up to date. Both ERα and ERβ consist of distinctive domains, such as N-terminal trans-activation domain, central DNA-binding domain (DBD), ligand-binding domain (LBD), and C-terminal coactivator/corepressor interacting domain (Zhao et al., 2008). Although the biochemical responses of ERα and ERβ to estrogens are largely similar in their modes, the two ERs show different and specific gene expression pattern in some tissues such as breast cancer (Thomas & Gustafsson, 2011; Haldosén et al., 2014).
In teleost fishes, ERα and ERβ are widely expressed in various tissues, and hepatic vitellogenesis which is a pivotal process for ovarian maturation provides a physiologically relevant endpoint of ER activation (Nelson & Habibi, 2013). In fact, hepatic vitellogenesis and the ERα gene expression are commonly used as bioassays for endocrine disruptors that have estrogen-like activity, whereas the biological significances of ERβ genes are not well elucidated up to now. Furthermore, involvement of ERβ in the control of estrogen-responsive genes in specific tissues is also poorly understood. In a previous report, E2-dependent induction of red fluorescent protein reporter gene expression driven by choriogenin H gene promoter was shown in transgenic marine medaka Oryzias dancena under a wide range of salinity conditions (Cho et al., 2013). To evaluate ERβ–mediated E2 responsiveness in euryhaline teleosts, we firstly investigated the expression profile of ERβ gene in several tissues and transcriptional activities of the ERβ proteins were examined in marine medaka.
MATERIALS AND METHODS
Total RNA was extracted from the whole body of adult medaka O. dancena, using the RNA Prep kit (Bioneer, Daejeon, Korea) according to the manufacturer’s protocol. To obtain full-length ERβ cDNA, the first-strand cDNA was synthesized with the extracted total RNA using a SMART rapid amplification of cDNA ends (RACE) cDNA Amplification kit (Clontech Lab, Palo Alto, CA, USA). A cDNA fragment was amplified with a forward and reverse primer set (#1 and #2; Table 1) based on known O. latipes and O. javanicus ERβ cDNA sequences (GenBank Accession nos. NM_001104702 and AY917148). PCR amplification was performed in a final volume of 20 μL containing 1 μg of the marine medaka whole-body cDNA, 2.5 mM dNTP 0.8 μL, 10 pmole of each oligo primer, 2× GC LA buffer 10 μL, 5U LA Taq DNA polymerase (Takara Bio, Shiga, Japan). The reactions were carried out under the following cycling conditions: 5 min denaturation at 94°C; 35 cycles of 30 s at 94°C, 30 s at 50°C, and 2 min at 72°C; and 7 min at 72°C. Full-length cDNAs for entire coding region of O. dancena ERβ (odERβ) were obtained by RACE PCR amplification in a final volume of 25 μL including 1 μg of 3’- or 5’-cDNA template, 2 pmole of gene-specific primers (#1 or #2; Table 1) and the Universal Primer A Mix (#3, Table 1), 10× Advantage 2 PCR buffer 2.5 μL, 10 mM dNTP mix 0.4 μL, and 50× advantage 2 polymerase mix (Clontech Lab) 0.4 μL with the following cycles: 5 min denaturation at 94°C; 5 cycles of 30 s at 94°C and 3 min at 68°C; 5 cycles of 30 s at 94°C, 30 s at 66°C, and 3 min at 72°C; 25 cycles of 30 s at 94°C, 30 s at 64°C, and 3 min at 72°C; and 7 min at 72°C. The amplified PCR products were cloned into pGEM-T Easy vector (Promega, Madison, WI, USA). To construct the odERβ expression vector, open reading frame of the odERβ cDNA was amplified by a conventional PCR using a primer set (#4 and #5, Table 1) and cloned into the Eco RI–Xho I restriction sites in pcDNA3-HA-NLS vector (Zavacki et al., 1997). Escherichia coli competent cells were transformed with the vectors and all of the constructs were sequenced by the Sanger method.
Underlined sequences indicate restriction enzyme recognition sites.
RACE, rapid amplification of cDNA ends.
The amino acid alignment was carried out by CLUSTALW with MEGA6 (Tamura et al., 2013), and the phylogenetic tree was constructed using the maximum-likelihood (ML) method based on the JTT matrix-based model (Jones et al., 1992). Branch supports were provided using 1,000 bootstrap replicates.
To examine tissue distribution pattern of ERβ1 transcripts, 12 kinds of tissues including brain, eye, fin, gill, heart, intestine, kidney, liver, muscle, spleen, ovary, and testis were surgically removed from mature marine medaka individuals (six males and six females; average body weight=2.3±0.3 g) that had been communally grown under the culture conditions: salinity at 15 ppt, temperature at 26±1°C and dissolved oxygen level at 6±1 ppm. Total RNA was extracted with Trizol reagent (Ambion, Thermo Fisher Scientific, Waltham, MA, USA) according to the manufacturer’s instruction and further purified with RNeasy Plus Mini Kit (Qiagen, Hilden, Germany) including the DNase I treatment step. Integrity and purity of total RNA sample was verified with 28S:18S rRNA ratio on formaldehyde/MOPS agarose gel and OD260/280 nm (260/230 nm) spectrophotometry, respectively. For end-point RT-PCR, equal amount of total RNA (1 μg) from each individual tissue was pooled within a tissue type, and an aliquot (2 μg) of the pooled RNA sample was reverse transcribed to cDNA using Omniscript RT kit (Qiagen) with oligo-dT20 priming method according to the manufacturer’s protocol. In order to prepare a normalized control (18S rRNA; GenBank accession number HM347347), an O. dancena 18S rRNA reverse primer (#6, Table 1) was also included in the RT reaction as described previously. RT product was 8-fold (for ERβ1) or 20-fold (for 18S rRNA) diluted with sterile water, and 2 μL of diluted cDNA was used as template for each PCR reaction. End-point RT-PCR was carried with a pair of primer (#7 and #8; Table 1) to amplify a 240-bp odERβ1 cDNA fragment. Thermal cycling conditions are 33 cycles of 94°C for 20 s, 58°C for 20 s and 72°C for 20 s with an initial denaturation step at 94°C for 2 min. From each cDNA template, a 253-bp fragment of 18S rRNA was also amplified as a normalization control with a pair of primer (#9 and #10; Table 1) under the same thermal cycling conditions described in Cho et al. (2011) except the reduction of cycle number to 22. Amplified products (5 μL) for both odERβ1 and 18S rRNA were electrophoresed on 2% agarose gels, and visualized with ethidium bromide (EtBr)-staining.
Based on the end-point RT-PCR assay, tissues examined to show the clear amplification of odERβ1 transcripts were selected for real-time quantitative RT-PCR (RT-qPCR). Basal expression levels of odERβ1 among those tissues were assayed with individual cDNA samples (three males and three females), and each cDNA template was assessed in triplicates. Oligonucleotide primers and thermal cycling conditions for odERβ1 and 18S rRNA were same as those used in end-point RT-PCR above, except the extension of the cycle number to 45. PCR was conducted with LightCycler 480 SYBR Green I Master Mix and LightCycler 480 Real-Time PCR System (Roche Applied Sciences, Penzberg, Germany). PCR efficiencies of both primer pairs (i.e., for odERβ1 and 18S rRNA) were 0.96 and 0.94, respectively. Basal expression level of odERβ1 transcripts in each tissue was determined with 2−ΔCT method based on the normalization against 18S rRNA level (Schmittgen & Livak, 2008).
HEK293 cells (5×104 cells/well) were routinely cultured in Dulbecco’s modified Eagle’s medium (DMEM) supplemented with 10% fetal bovine serum (FBS, HyClone, GE Healthcare, Chicago, IL, USA) and 1% antibiotic-antimycotic (Invitrogen, Grand Island, NY, USA) in a humidified atmosphere containing 5% CO2 at 37°C. The cells were seeded in 24-well plates and transfected with odERβ1, O. latipes ERβ1 (olERβ1), and masu salmon ERα (msERα) expression vectors and reporter vectors including an estrogen-response element (ERE)-driven luciferase reporter (ERE-Luc) (Maeng et. al., 2005), and internal control vector for β-galactosidase (200 ng each) using LipofectamineTM 2000 (Invitrogen). The olERβ expression vector was kindly provided by Dr. Yoshitaka Nagahama, National Institute for Basic Biology, Okazaki, Japan. After 3 hr post-transfection, the cell medium was changed by DMEM with 10% charcoal-stripped FBS and 1% antibiotic-antimycotic. Detailed transfection, ligand treatment and luciferase assay were conducted as described previously (Maeng et al., 2005).
HEK293 cells were seeded on poly-D-lysine hydrobromide (Sigma-Aldrich, St. Louis, MO, USA)-coated coverslips and transiently transfected with HA-tagged ER expression vectors (200 ng each) as described before. After 30 h, the cells were fixed for 10 min in 4% paraformaldehyde in PBS (Wako, Osaka, Japan) and further incubated for 10 min with PBS containing 0.1% Triton X-100. To block non-specific binding, the cells were incubated with 1% bovine serum albumin in PBST (PBS+0.1% Tween 20) for 30 min at room temperature. The cells were incubated with a 1:5,000 dilution of monoclonal anti-HA antibody produced in mouse (H9658, Sigma-Aldrich) in PBST overnight at 4°C in a humidified chamber. The cells were further incubated with a 1:2,000 dilution of anti-mouse IgG (H+L), F(ab’)2 Fragment (AlexaFluor 488 conjugate, Cell Signaling, Danvers, MA, USA) in PBST for 1 h at room temperature in the dark. The coverslips were mounted with Fluoroshield Mounting Medium with DAPI (Abcam, Cambridge, UK) and the cells were observed using a fluorescence microscope (Eclipse E200, Nikon, Tokyo, Japan). Fluorescence signals were not detected in the control cells incubated without the primary antibody.
Differences in basal mRNA expression levels of odERβ among adult tissues and luciferase activities determined with reporter assays for ERs were assessed with ANOVA followed by Duncan’s multiple range test, while differences between sexes given a tissue type with Student’s t-test. Difference was considered to be significant when p<0.05.
RESULTS
We cloned an ERβ1 cDNA from the whole body of adult marine medaka O. dancena based on O. latipes and O. javanicus ERβ cDNA sequences. The cDNA contained an open reading frame of 1,560 nucleotides encoding 519 amino acid residues. The overall protein sequence identities of the odERβ1 was relatively higher with ERβ1 subtype of O. latipes, Paralichthys olivaceus, and Oncorhynchus mykiss (93.8%, 81.5%, and 69.4%, respectively), but relatively lower with ERβ2 and ERα subtypes of teleosts and human (44.1%–57.0%; Fig. 1A). Amino acid sequences of N-terminal A/B and DNA binding C domains of odERβ1 were strikingly similar to those of olERβ1 (96.1% and 100%), while the sequences of hinge D domain and ligand binding E/F domains of odERβ1 showed relatively lower similarities with the corresponding regions of olERβ1 (82.9% and 92.3%). The C-terminal amino acid sequence of odERβ1 was short when compared with those of olERβ1 and human ERβ (Fig. 1B). A molecular phylogenetic analysis revealed that teleostean ERβ1 proteins including odERβ1 formed a monophyletic group that was distinct from a clade comprising tetrapodian ERβ and teleostean ERβ2 and ERα proteins (Fig. 2).
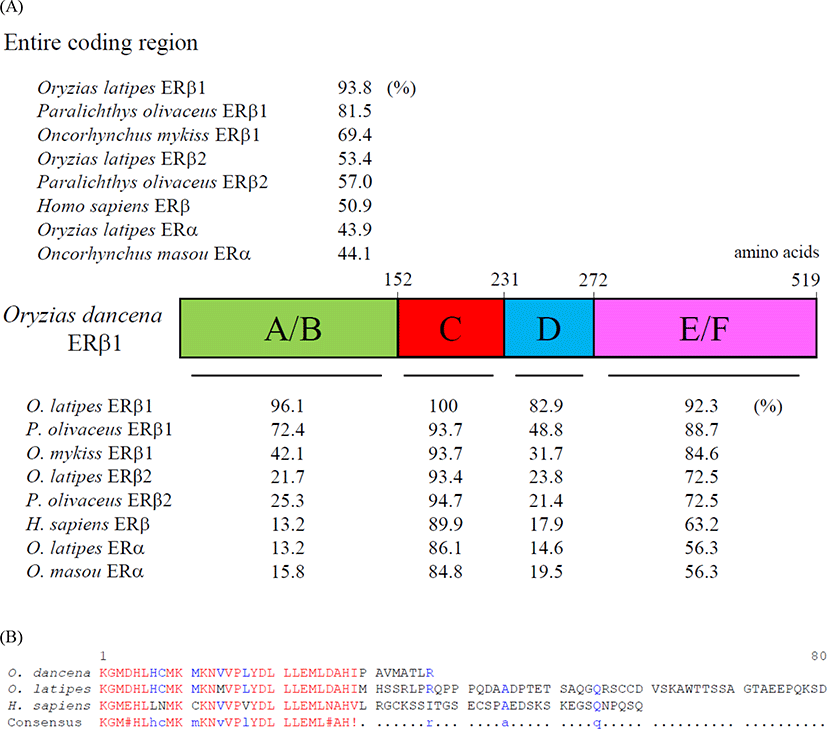
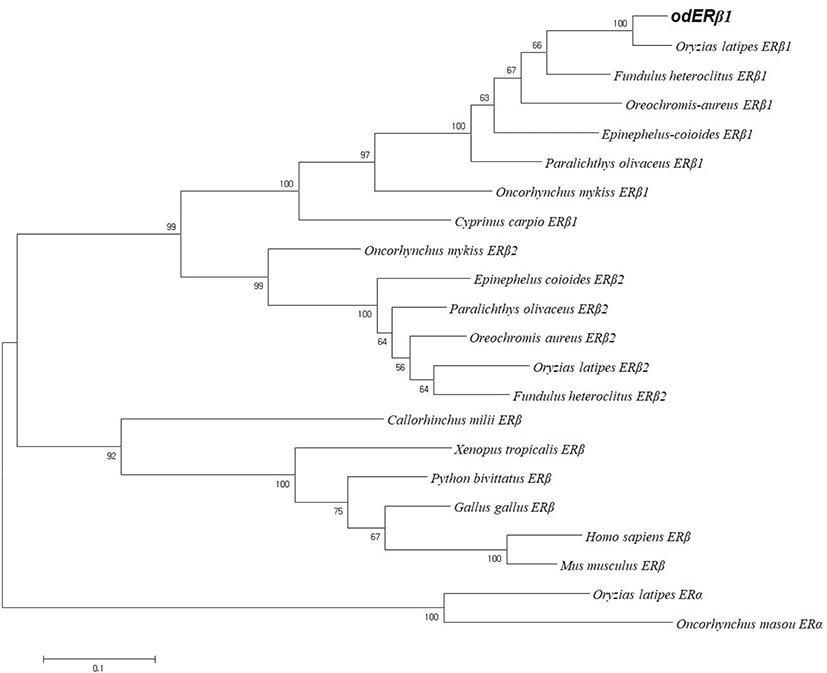
From end-point RT-PCR analysis, odERβ1 mRNAs were predominantly expressed in the ovary (Fig. 3A). Besides the ovary, odERβ1 transcripts were also detectable in the brain, gill, intestine, kidney, and muscle, although expression levels in those tissues were significantly lower than that of the ovarian expression. Meanwhile, under the present RT-PCR conditions, odERβ1 mRNAs were not clearly detected in the eye, fin, heart, liver, spleen and testis. The robust expression of odERβ1 mRNAs in the ovary was clearly reproduced in RT-qPCR assay with female tissues, in which the ovarian expression level was 3.8–15.3 times greater than those in other tissues examined (p<0.05; Fig. 3B). Among non-ovarian tissues, brain showed a higher expression compared to other tissues (p<0.05). Expression patterns in male somatic tissues were not significantly dissimilar from those observed in female. Like in female tissues, male brains displayed a relatively higher expression than others did, although the difference was not highlighted as much as in female (Fig. 3C). In comparison between genders within a given tissue, females tended to show a higher expression level of odERβ1 in the brain than males did, however, the difference was not statistically significant due to large individual variations. There was no gender-dependent difference in the expression level of the expression level of odERβ1 for gill, intestine, muscle and kidney (p>0.05).
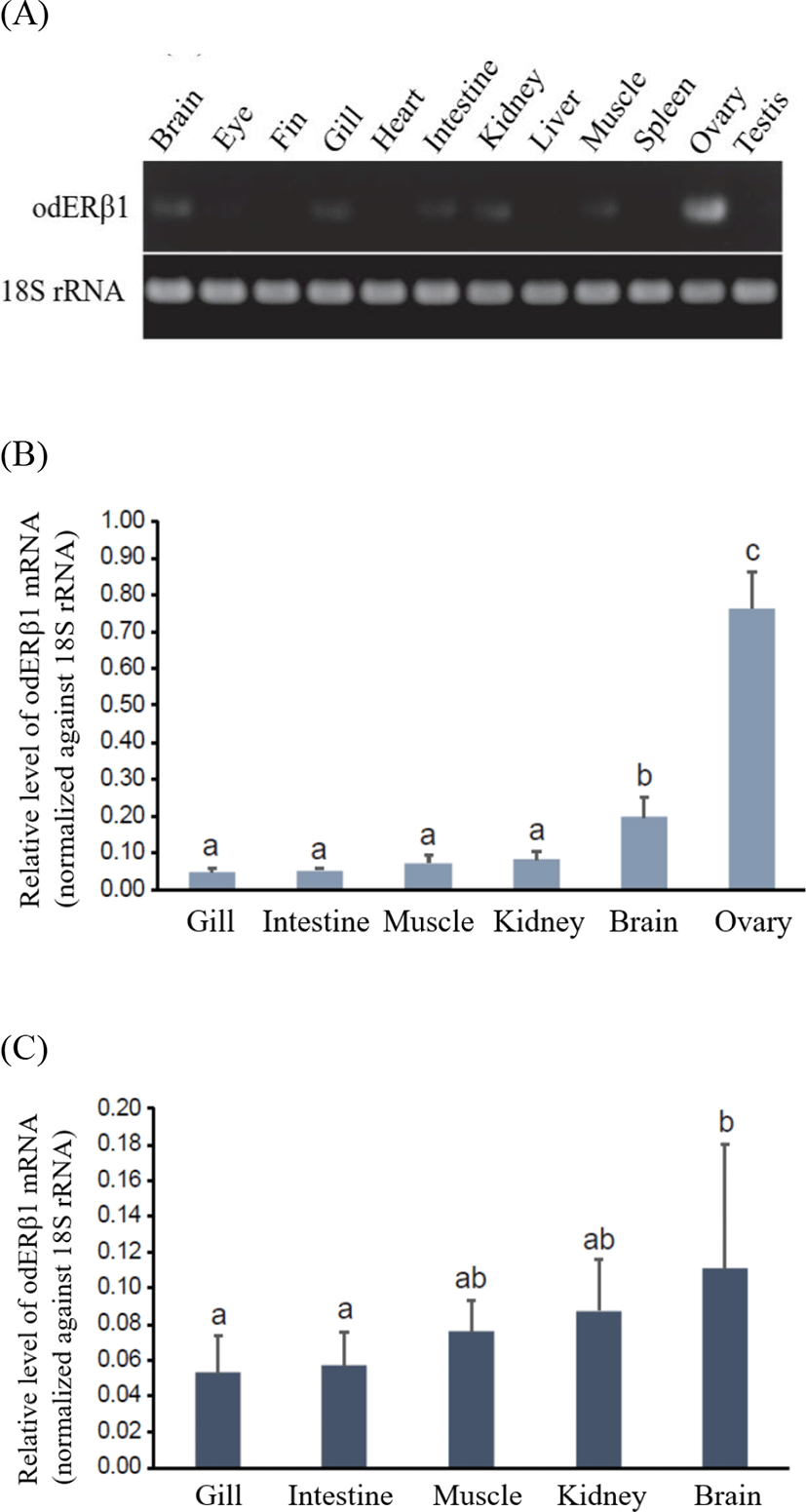
The transcriptional activity of the odERβ1 was evaluated by cotransfection of a luciferase reporter, ERE-Luc, into a mammalian cell line, HEK293. The ERE-Luc reporter activities of odERβ1 were significantly elevated by E2 and the response of odERβ1 showed a dose dependent manner (p<0.05). The transcriptional fold-activation of odERβ1 in the presence of E2 was markedly weak, when the activities of O. latipes ERβ1 (olERβ1) and Oncorhynchus masou ERα (msERα) were compared (Fig. 4B). The fold-transactivation of odERβ1 by E2 treatment showed a dose dependent manner. Transfected HA-tagged odERβ1, olERβ1, and msERα expression vectors were mainly expressed in the nucleus of HEK293 cells (Fig. 4C).
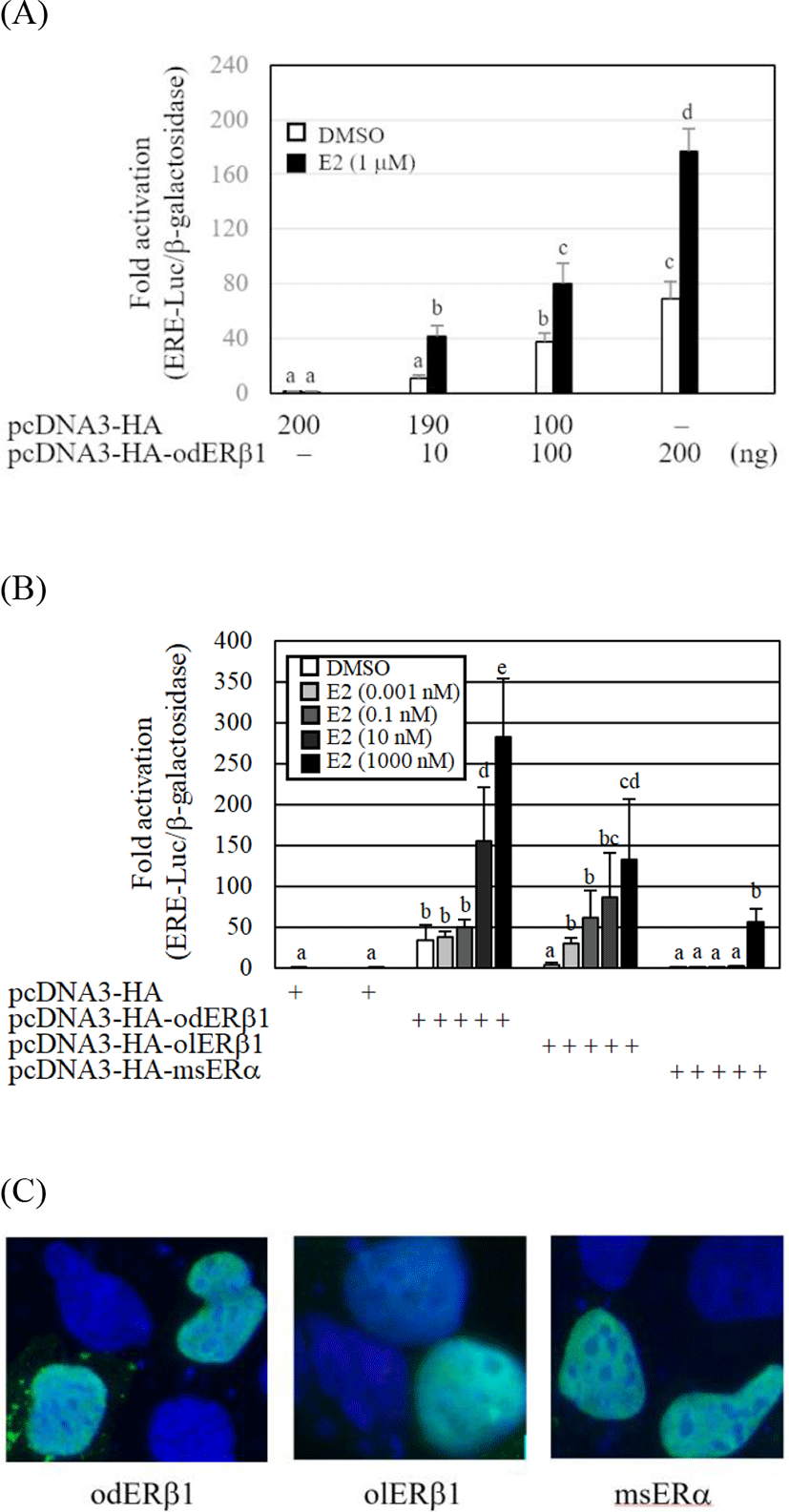
DISCUSSION
In the present study, we cloned an ERβ1 subtype cDNA from O. dancena and examined the mRNA expression profile in various tissues. To date, two ERβ subtypes (ERβ1 and ERβ2) have been isolated from a variety of teleost fish species, including Japanese medaka and largemouth bass (Sabo-Attwood et al., 2004; Chakraborty et al., 2011), although a single ERβ gene was reported in mammals (Haldosén et al., 2014). The overall sequence identity analysis of three subtypes of ER indicated that odERβ1 is the ortholog of teleost ERβ1. This classification was supported by a phylogenetic analysis: ERβ1 and ERβ2 subtypes of teleost species and ERβ of tetrapods. The estimated amino acid length of odERβ1 were 519 which is shorter than olERβ1 (562 amino acids; Chakraborty et al., 2011) and human ERβ (530 amino acids; Ogawa et al., 1998). The lacking of large portion of F domain known as a hormone-dependent activation function AF-2 (Glass & Rosenfeld, 2000) is the most different feature between odERβ1 and olERβ1. Taken together, odERβ1 is most likely a splicing variant of ERβ1 gene. In human, multiple ERβ isoforms lacking or changing of F domain by alternative splicing of the last coding exons have been reported (Haldosén et al., 2014). The AF-2 region of E2-bound ERs mainly interact with transcriptional co-regulators, i.e., N-CoR, SMRT, and GRIP1, and then the ER complex may recruit the basal transcription machinery including RNA polymerase II (Glass & Rosenfeld, 2000; Webb et al., 2003). Although the function of AF-2 region of ERβ is unclear in teleosts, AF2-deleted female mice were significantly less preferred by adult males than that of normal females in odor preference tests (Antal et al., 2012). Implication of ERβ1 variants in the reproductive axis of teleosts is needed to be elucidated.
Tissue-specific expression of ER subtypes was demonstrated during the life cycle of teleosts (Sabo-Attwood et al., 2004; Chakraborty et al., 2011). The odERβ1 transcripts were strongly expressed in the ovary and significantly detectable in the brain than those of gill, intestine, kidney, and muscle, whereas olERβ1 mRNA expression was observed as faint bands in the testis and the brain (Chakraborty et al., 2011). In rainbow trout, the mRNA for ERβ1 was detected in diverse tissues, e.g., the testis, ovary, brain, and kidney (Nagler et al., 2007). Interestingly, the rainbow trout ERβ1 transcript abundance in the ovary showed the highest expression at the beginning of the reproductive cycle, but then decreased afterward (Nagler et al., 2012). The expression of rockfish ERβ1 mRNA was also significantly higher in the ovary at the early-oocyte stage (Mu et al., 2013). The brain of female O. dancena showed relatively higher level of odERβ1 transcript than other tissues examined. Recently, ERβ1-null female medaka showed apparently normal sexual behavior, but without fertility and oviposition in response to male courtship (Kayo et al., 2019). These observations suggest that ERβ1 expression may be important for estrogen signaling that starts the next cycle of ovarian development through brain-pituitary-gonad axis in teleosts. In fact, expression of esr1 (ERα gene), gnrhr1, fsh, lh genes in the brain of red spotted grouper was positively associated with the formation of ovarian cavity (Kim et al., 2016). More in-depth investigations of individual tissues during different reproductive stages of medaka O. dancena will be required to better understand the biological significance of ERβ isoforms.
The transcriptional activities of odERβ1 were significantly elevated by E2 concentration dependent manner. In addition, the nuclear localization and the E2-dependent transactivation mode of odERβ1 were evidently demonstrated like those of olERβ1 and msERα. These results are similar to a previous report that three medaka ER subtypes including olERβ1 are capable of initiating transactivation of vitellogenin genes via upstream EREs (Lee Pow et al., 2016). The transcriptional activity of steroid hormone receptor superfamily including ERs is mainly dependent upon C-terminal ligand-binding E/F domain and AF-2 region (Glass & Rosenfeld MG, 2000). When the amino acid sequence of odERβ1 was compared with those of olERβ1 and human ERβ, odERβ1 was estimated to be a splicing variant from ERβ1 gene that lacks F domain and/or AF2 region. The olERβ1 having a long F domain showed higher fold-activation of transcription of ERE-driven reporter gene in the presence of E2, whereas odERβ1 showed relatively weak fold-activation of the reporter gene in the presence of E2. These data indicate that the complete F domain and AF2 region are important for ligand binding and direct E2-dependent transcriptional activity of ERβ1. In fact, a mutant mammalian ERα deleted for the AF2 core domain impairs E2-induced transactivation by wild type ERα (Jung et al., 2001).
In summary, we cloned an O. dancena ERβ1 variant having a short C-terminal activation function 2 region. The odERβ1 mRNAs were highly expressed in the ovary and brain. The transcriptional activity of odERβ1 increased by E2 in a dose dependent manner, although E2-dependent transactivation of odERβ1 was weaker than that of O. latipes ERβ1.