INTRODUCTION
Melatonin (N-acetyl-5-methoxytryptamine, MEL), a neuroendocrine hormone synthesized and secreted from the pineal gland of the brain, plays a significant physiological role in the regulation of fish gonadal development (Tosini & Menaker, 1996; Borjigin et al., 1999; Mondal et al., 2017). In vertebrates, including teleosts, plasma MEL, and photoperiod-dependent neurohormones in fish (Goldman, 2001; Reiter et al., 2009), levels are rhythmically expressed through a 24 h light-dark cycle, as the plasma MEL concentration is low during the daytime and high during the nighttime (Falcón et al., 1987; Bolliet et al., 1996; Migaud et al., 2010; Ogiwara & Takahashi, 2016).
MEL mediates the relationship between circadian rhythms and the hypothalamic-pituitary-gonadal (H-P-G) axis, thereby regulating reproductive events, including oocyte development and maturation (Vera et al., 2006; Falcón et al., 2007, 2010; Tamura et al., 2014). In other words, the pineal gland translates environmental signals, such as the photoperiod, into rhythmic changes in hormonal fluctuations in the H-P-G axis; photoreceptor cells of the pineal gland produce MEL in a day-length-dependent manner (Falcón et al., 2007; Brüning et al., 2018). MEL receptors are distributed in all brain areas, including the pituitary gland, retina, and many other tissues, such as the ovary (Falcón et al., 2010; Hong et al., 2014). These studies suggested that MEL exerts direct effects on teleost ovaries by activating MEL receptors. MEL acts directly at the ovarian level to modulate ovarian physiology, including steroidogenesis (Adriaens et al., 2006; Wang et al., 2019), folliculogenesis (Choi et al., 2015), oocyte maturation (Maitra et al., 2013) and ovulation (Ogiwara & Takahashi, 2016). In medaka ovaries, Ogiwara & Takahashi (2016) reported that pre-ovulatory follicles are capable of synthesizing MEL. They found that MEL is constitutively synthesized in the granulosa cells of preovulatory follicles during the 24 h spawning cycle of fish (Takahashi & Ogiwara, 2021). Moreover, MEL has been detected in fish ovaries (Hasan et al., 2014).
Therefore, the direct effects of MEL on the gonads of fish were supported. Chattoraj et al. (2005, 2008) showed that pre-incubation with MEL accelerated the action of maturation inducing hormone (MIH) during oocyte maturation; 17α,20β-dihydroxy-4-pregnen-3-one (17α20βP) has been considered to be MIH in most teleost fish (Patiño & Sullvian, 2002; Nagahama & Yamashita, 2008). Similarly, the influence of MEL on the process of oocyte maturation has been reported in zebrafish; exposure of ovarian follicles to MEL significantly increased the rate of germinal vesicle breakdown (GVBD) in oocytes of MIH-treated follicles (Carnevali et al., 2011). Incubation of oocytes with MEL diminishes the stimulatory effect of MEL and MIH on the rate of GVBD (Maitra & Hasan, 2016). However, the specific mechanisms by which MEL directly regulates reproductive events remain unclear.
Therefore, we examined the direct effects of exogenous MEL on longchin goby oocytes at different stages of maturation (pre-, mid-, and late-vitellogenesis) by investigating the GVBD rate in vitro. In addition, we examined whether preincubation with MEL accelerates MIH-induced maturation. These trials were performed based on the previously reported ranges of circulating MEL levels (Vera et al., 2006; Nikaido et al., 2009; Oliveira et al., 2011).
MATERIALS AND METHODS
Experimental fish (body length: 5.1–6.5 cm) were captured from tide pool at Cheong-sa-po, Busan, Korea, during reproductive period (March to May).
Ovaries were dissected from several mature females, rinsed with ice-cold balanced salt solution (132.96 mM NaCl, 3.09 mM KCl, 0.28 mM MgSO4 · 7H2O, 0.98 mM MgCl2 · 6H2O, 3.40 mM CaCl2 · 6H2O, and 3.65 mM HEPES), and separated into different maturation stages according to the diameters measuring with an ocular micrometer under a dissecting microscope. The extracted ovaries were categorized into three different maturation stages: pre-vitellogenesis (0.61–0.66 mm of oocyte diameter), mid-vitellogenesis (0.71–0.74 mm of oocyte diameter), and late-vitellogenesis (0.80–0.86 mm of oocyte diameter) (Baek et al., 2007).
Oocytes were incubated in each well of 24-well culture plates containing 1 mL of Leibowitz L-15 medium (Gibco, Grand Island, NY, USA) with (a) MEL at the concentrations of 5, 10, 50, 100, 500, and 1,000 pg/mL, (b) 50 ng/mL of 17α20βP which is MIH of this species, or (c) both 50 ng/mL of 17α20βP and MEL (4 h pre-incubated before addition of 17α20βP, at the concentration 50, 100, and 500 pg/mL). The pH and osmolarity of the media were adjusted to 7.7 and 300 mOsm, respectively. Each plate was cultured for different incubation times of 24 h (mid- and late-vitellogenesis) or 48 h (pre-vitellogenesis) at 18°C with constant gentle shaking. Each treatment was conducted in three wells with 20 oocytes per well and all experiments were repeated three times.
At the end of the incubation period, oocytes were fixed with a clearing solution (ethanol: formalin: glacial acetic acid=6:3:1). The effects of the treatments on oocyte maturation were determined by GVBD using a dissecting microscope (BX-50, Olympus, Tokyo, Japan).
RESULTS
Oocytes isolated from the ovaries of the longchin goby are shown in Fig. 1. Each developmental stage of oocytes was observed under a microscope immediately before incubation. In pre- and mid-vitellogenic oocytes, GVs were located in the middle of the cytoplasm (Fig. 1A and B). GVs of late-vitellogenic oocytes were still in the middle of the cytoplasm, and migrating GVs were not observed (Fig. 1C).
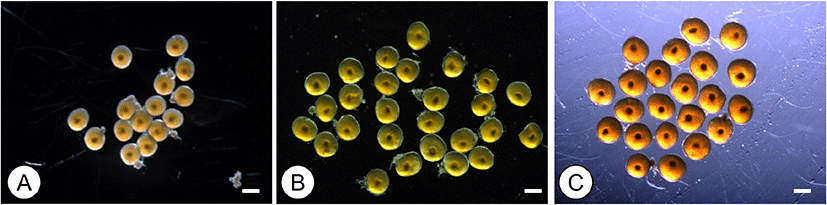
We investigated the direct effects of exogenous MEL on longchin goby oocytes at various stages of maturation. As shown in Figs. 2–4, MEL did not show any significant effect on GVBD, except for one experiment in 0.74 mm diameter oocytes were cultured with 10 pg/mL MEL (p<0.05).
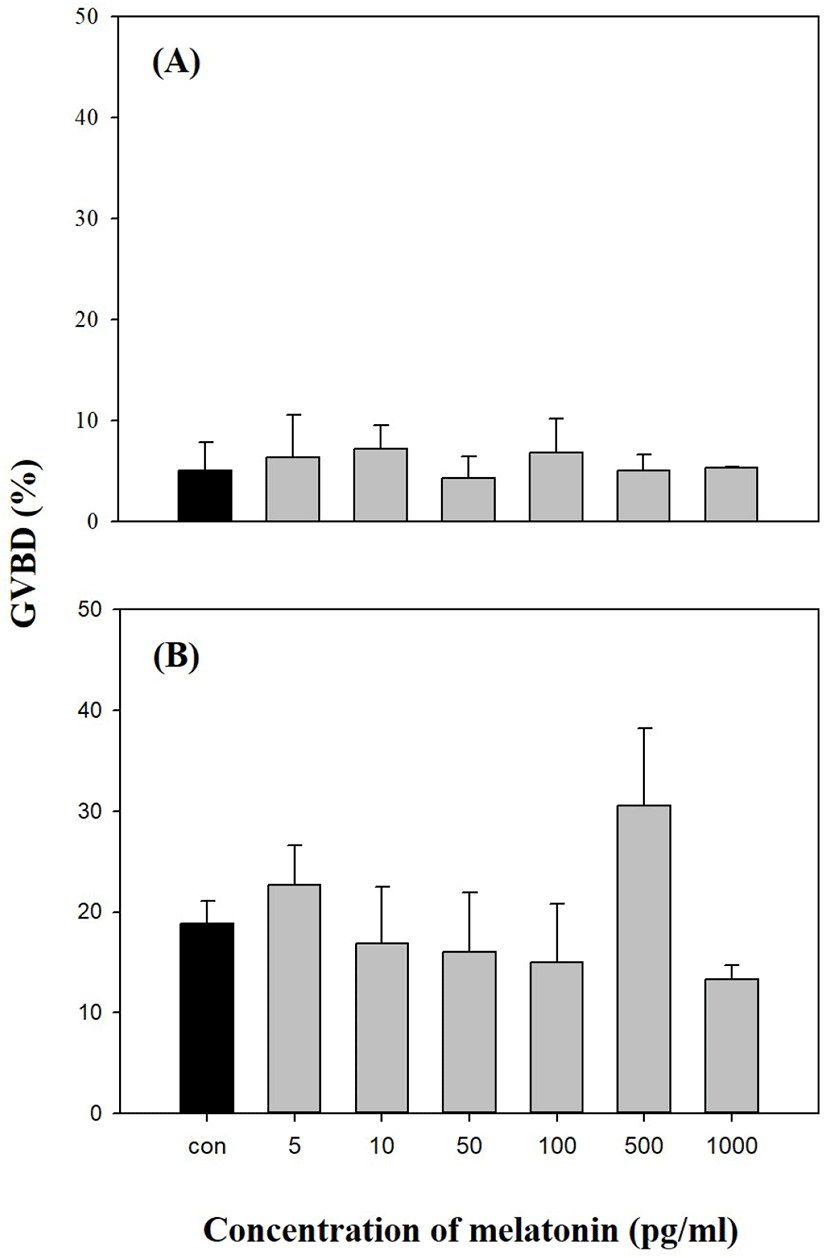
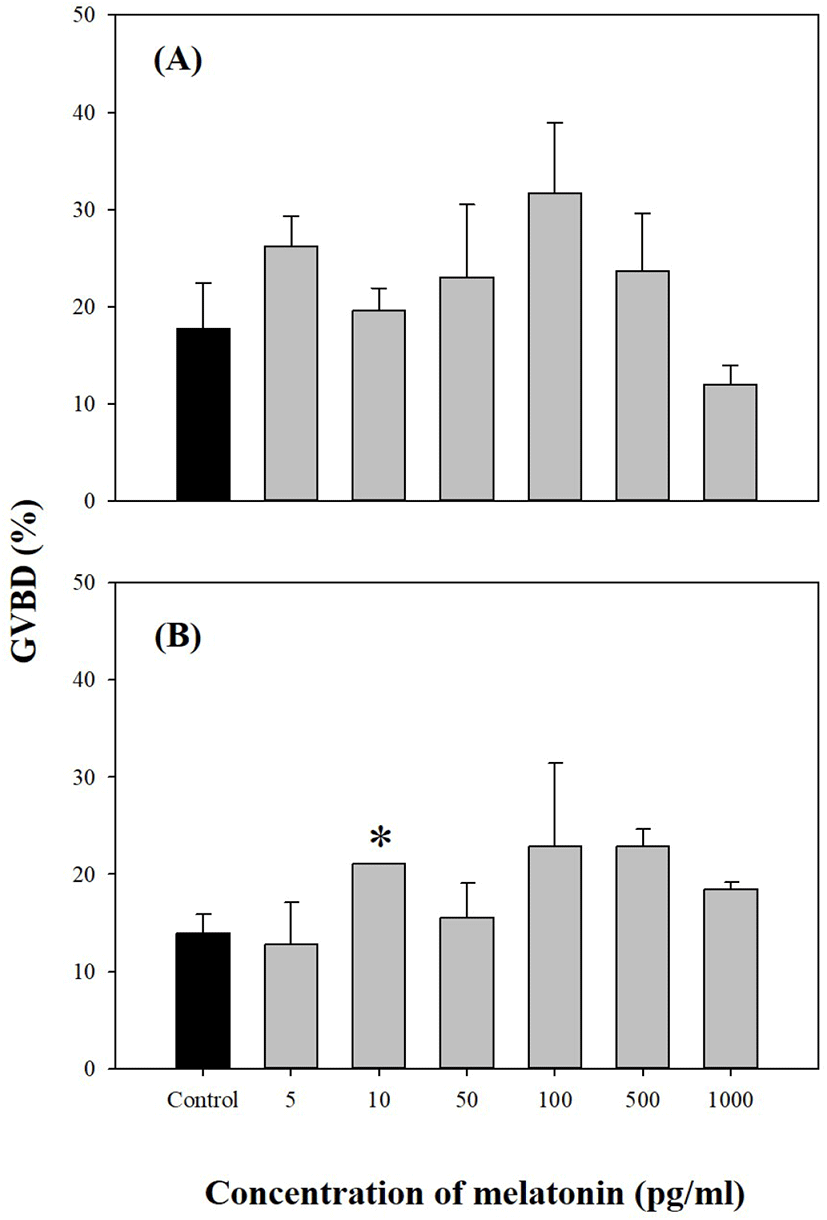
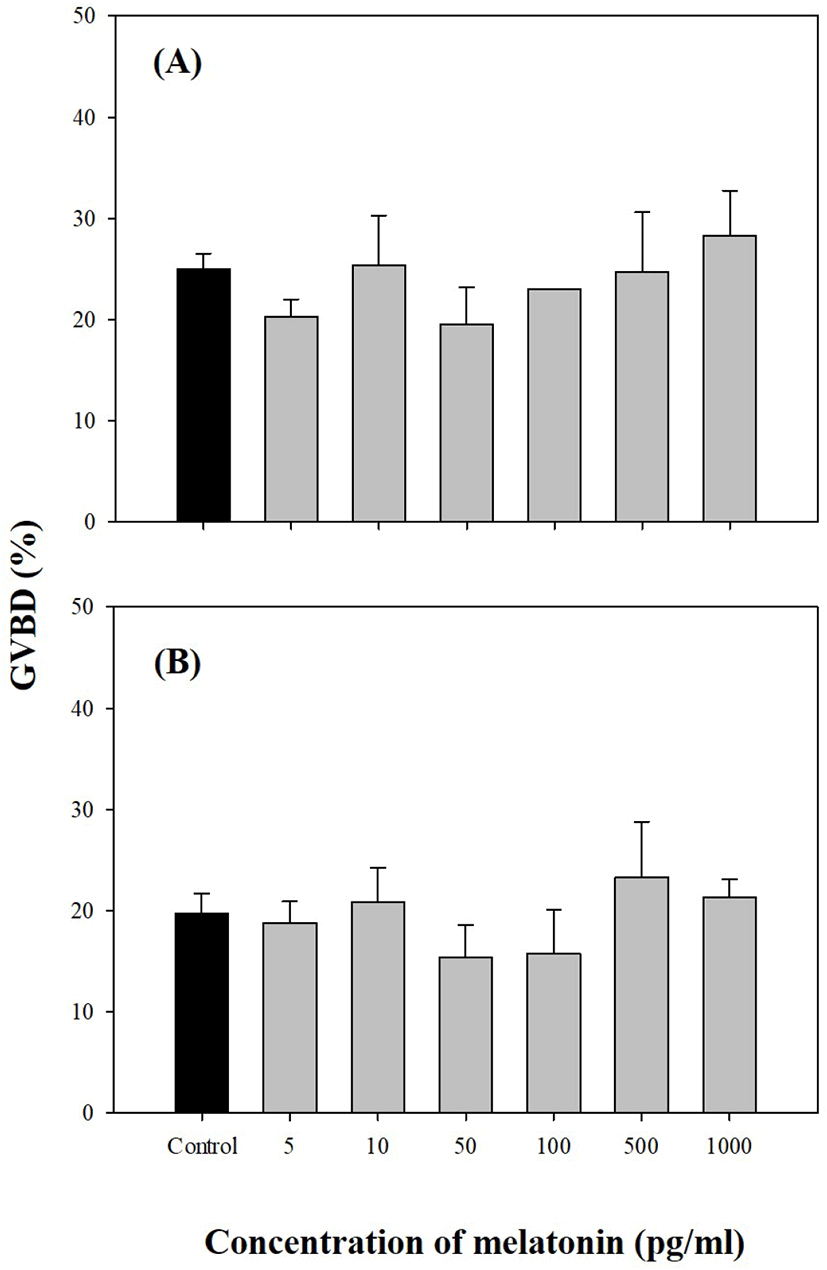
Oocytes of late-vitellogenesis stage (0.80 and 0.86 mm) were incubated with 50, 100, and 500 pg/mL MEL alone or in combination with 50 ng/mL MIH (17α20βP; Fig. 5). Significant GVBD induction was observed with 50 ng/mL MEL combined with 50 ng/mL MIH (p<0.05). Other higher levels of MEL treatment (both 100 and 500 pg/mL) with 50 ng/mL MIH showed an increase in GVBD compared to single treatment with 50 ng/mL MIH, although the difference was not significant.
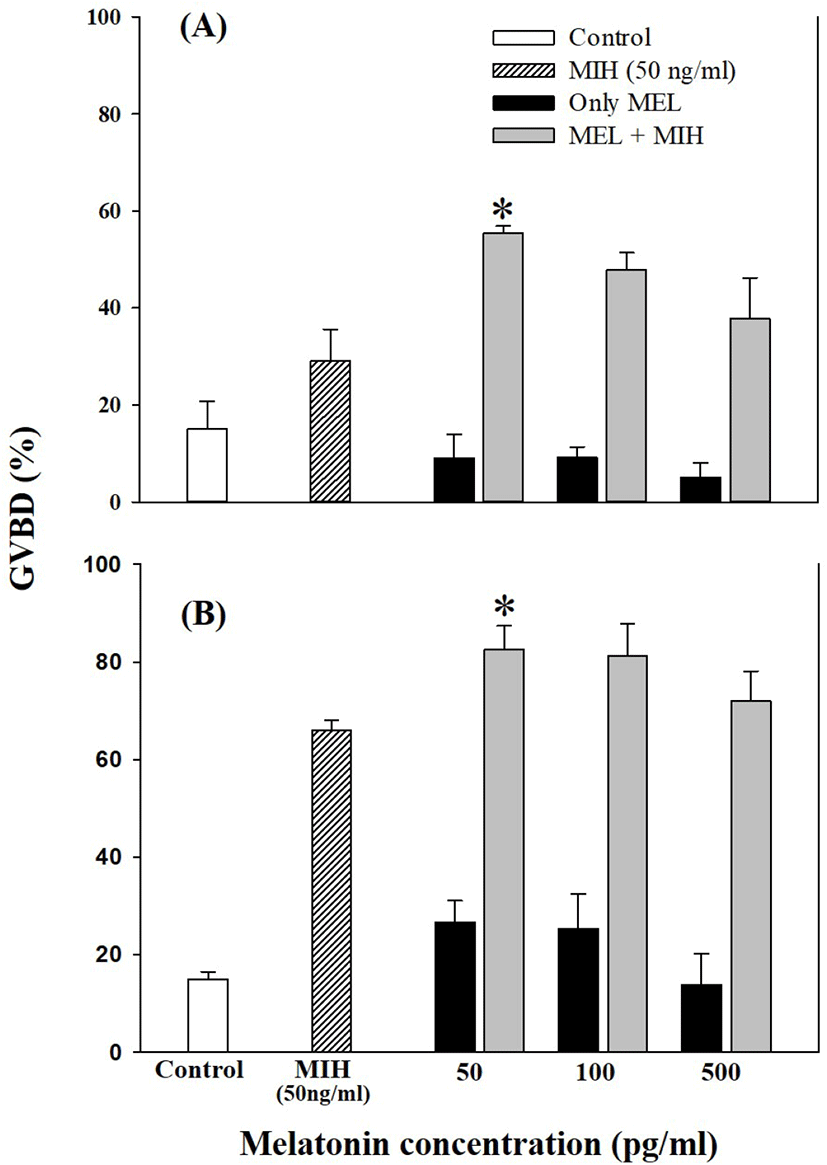
DISCUSSION
A recent review by Takahashi & Ogiwara (2021) showed that MEL, a neurohormone primarily synthesized and secreted in the pineal glands of vertebrates following a circadian rhythm, is an important regulator of various physiological processes, including reproduction. Moreover, in teleosts, this hormone is synthesized in the ovary and acts directly at the ovary level to regulate ovarian physiology, including steroid hormone synthesis, oocyte maturation, and ovulation (Maitra & Hasan, 2016; Mondal et al., 2017).
In teleosts, MEL has been detected in the ovaries of Indian carp (Calta catla) (Hasan et al., 2014; Mondal et al., 2017), medaka (Oryzias latipes) (Ogiwara & Takahashi, 2016), and zebrafish (Danio rerio) (Yumnamcha et al., 2017; Khan et al., 2018). In these studies, serum MEL levels were low during the day and high at night. MEL circulating levels range from 146.6 to 697.7 pg/mL in seabream (Diplodus puntazzo) (Vera et al., 2006), 69 to 224 pg/mL in Mozambique tilapia (Oreochromis mossambicus) (Nikaido et al., 2009), and 34.1 to 287.6 pg/mL in Senegal sole (Solea senegalensis) (Oliveira et al., 2011), even though reared conditions are different, under various light and temperature conditions. Based on these levels, our experiments set concentrations in the range of 5–1,000 pg/mL in vitro. Based on the in vitro study of Chattoraj et al. (2005), the pre-incubation time of MEL was set to 4 h. Incubation time of goby oocytes was set to 24–48 h according to our previous studies (Baek et al., 2007; Baek, 2008).
In this experiment, the direct treatments of MEL alone at concentrations of 5, 10, 50, 100, 500, and 1,000 pg/mL in vitro using the oocytes of pre-vitellogenesis (0.61–0.66 mm diameter), mid-vitellogenesis (0.71 and 0.74 mm diameter), and late-vitellogenesis (0.81 and 0.86 mm diameter) did not evoke any response to GVBD except 0.74 mm diameter oocytes cultured with 10 pg/mL MEL (p<0.05). This could be due to differences in the developmental stages of the oocytes. We suspect that the oocytes of 0.74 mm were more sensitive to the action of MEL than the oocytes of other sizes, and the extent of the stimulatory effect on GVBD depends on MEL. However, the combination of MEL and MIH in late-vitellogenesis (0.81 and 0.86 mm) oocytes increased the rate of GVBD compared to other experimental groups (treated with MIH or MEL alone); 50 pg/mL MEL had maximum acceleratory effect on MIH induced GVBD of oocytes. These results indicated that the activity of MIH-induced GVBD was accelerated after 4 h of pre-incubation with MEL. Similar results regarding the direct effects of MEL on fish oocyte maturation, including GVBD, have been reported by Chattoraj et al. (2005), who showed that pre-incubation with MEL accelerated the action of MIH during oocyte maturation in carp (Labeo rohita and Cyprinus carpio). Similarly, during oocyte maturation in zebrafish, the exposure of ovarian follicles to MEL significantly increased the GVBD rate of MIH-treated follicles (Carnevali et al., 2011). Conversely, the incubation of oocytes with MEL diminished the stimulatory effect of MEL and MIH on the rate of GVBD in Catla catla (Chattoraj et al., 2008; reviewed by Maitra & Hasan, 2016).
Exogenous MEL treatment leads to elevated intraovarian MEL titers, which may stimulate endogenously available antioxidants to reduce the oxidative stress in the carp ovary and accelerate (during the preparatory phase) or retard (during the pre-spawning or spawning phase) the growth of oocytes (Mondal et al., 2017). Takahashi & Ogiwara (2021) reported that the direct action of MEL affects ovarian steroidogenesis in some teleosts; for example, using a mudskipper with fully grown ovarian follicle during the spawning season, plasma 17α20βP levels were significantly increased after MEL injection (Hong et al., 2014). They also showed that MEL directly stimulated ovarian fragments in vitro to produce significantly higher progestin levels. Takahashi & Ogiwara (2021) suggested that MEL affects oocyte maturation via its antioxidant activity. However, the molecular mechanisms underlying the direct effects of MEL remain largely unknown.
Carps treated with MEL at various phases of the reproductive cycle showed correlations among ovarian MEL, different antioxidants, and different stages of oocyte growth (Mondal et al., 2017). Further research is needed to prove the roles of MEL, both in its direct action on the ovary and its indirect effects via the H-P-G axis, in fish reproduction.